March 24, 2021 feature
Understanding the structural and chemical heterogeneities of surface species at the single-bond limit
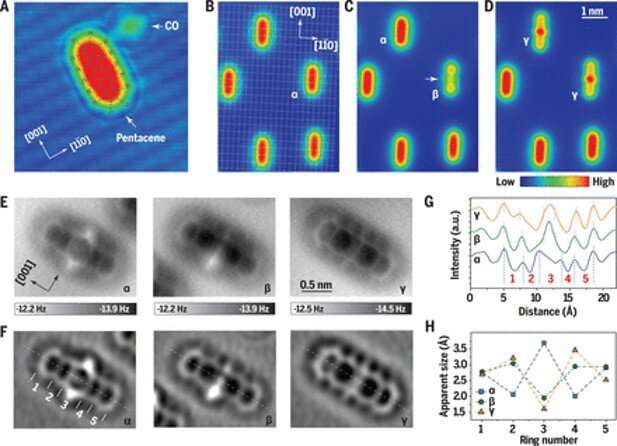
Advances in tip-based microscopy in materials science have allowed imaging at angstrom-scale resolution, although the technique does not provide clear characterization of the structural and chemical heterogeneities of surface species. In a new report now published on Science, Jiayu Xu and a research team in quantum information and quantum physics at the University of Science and Technology of China used a model system of pentacene derivatives on a silver surface. The researchers then combined a range of materials characterization techniques including scanning-tunneling microscopy, atomic force microscopy and tip-enhanced Raman scattering to provide electronic, structural and chemical information to characterize diverse, yet structurally similar chemical species relative to their interaction with the metal surface at single-bond resolution. The proposed multi-technique approach has wide applications across fundamental studies for heterogenous catalysis of surface chemistry.
Joint strategy for surface chemistry
Molecules that are adsorbed on a surface can undergo marked changes to form different surface species as a result of structural defects, chemical bond breaking and/or chemical bond formation. Materials scientists are keen to identify the structure or heterogeneity of surface species to better understand surface science. Such efforts require precise characterization of chemical bonds within molecules and substrates. Researchers have used a variety of tip-based microscopic and spectroscopic methods to achieve the task including scanning-tunneling microscopy (STM), scanning-tunneling spectroscopy (STS) and noncontact atomic force microscopy (AFM), to resolve static electronic structures and intramolecular geometric surface species maintaining high energy and resolution. The techniques are limited due to a lack of chemical sensitivity, which can hinder its ability to determine the heterogeneity (diversity) of surfaces. To overcome the weakness, researchers have used tip-enhanced Raman spectroscopy (TERS). Based on the method, scanning Raman picoscopy (SRP) provided an optical method with single-bond resolution to fully map individual vibrational modes and visually develop chemical structures of single molecules. All three methods can reach an angstrom-level resolution in real space, a combination of these methods can provide comprehensive detail to interrogate the heterogeneity of surface species. Xu et al. first selected pentacene (C22H14) on the silver surface as the model system. Pentacene is a benchmark system often used to characterize the resolution and performance of STM and AFM techniques.
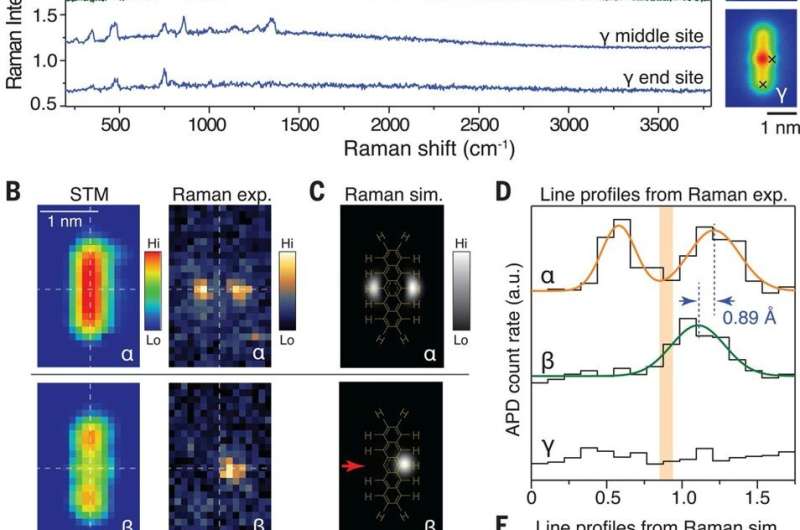
The experiments
During this work, the team obtained STM (scanning-tunneling microscopy) images of an anatomically resolved metal surface with adsorbed pentacene and carbon monoxide (CO) molecules at a low voltage bias. When the team applied voltage pulses of 2.0 V on to a molecule, they formed two kinds of new species with different shapes. These included species β with a dumbbell-like shape and species γ with a spindle-like shape. Pentacene and its derivatives also strongly showed voltage-dependent contrast in the STM topographies alongside different electronic states in the STS spectra. The plasmonic excitation of the system appeared to be strongly responsible for the transformation of pentacene. The use of STM and STS (scanning-tunneling microscopy and scanning-tunneling spectroscopy) alone could not directly determine the actual chemistry of the transformed species. As a result, Xu et al. used AFM (atomic force microscopy) with a CO-decorated tip to further understand the three species, which include the intact pentacene molecule (α). They noted the appearance of dark halos, arising from van der Waals attraction at the periphery of all three species (α, β, γ) where the inner molecular structures maintained atomic resolution, which originated from short range Pauli repulsion. The AFM method provided more structural detail compared to STM. The work showed how the center of carbon atoms of pentacene could interact with the silver surface. Xu et al. noted interactions between two possible sources of transformation from α to γ, allowing the central benzene ring to open with atom rearrangements; to confirm either hypothesis, they needed to know more about local chemical bonds.
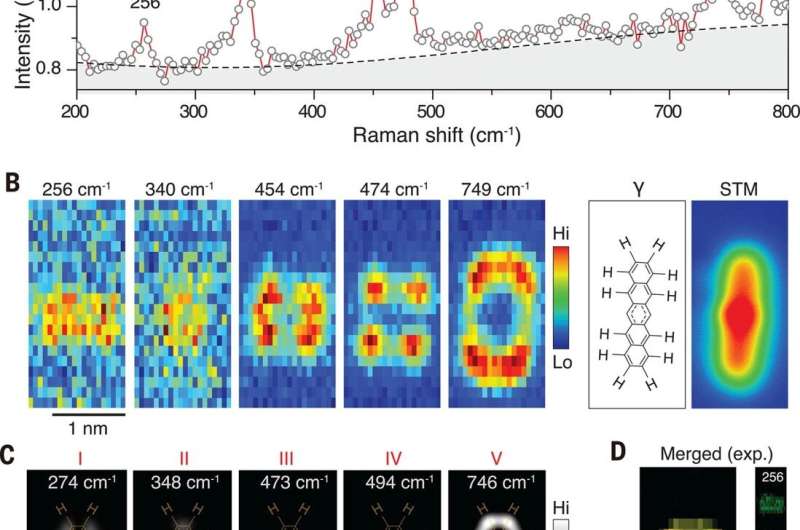
Understanding chemical bonds
The researchers used TERS (tip-enhanced Raman spectroscopy) measurements to characterize chemical bond information—since the Raman signals were directly related to the vibrational motion of the bonds. The team obtained Raman spectra from species α, β and γ by locating the tip across the sites of the sample. The carbon-hydrogen (C-H) stretching mode of pentacene appeared alone in the high-wave number region to provide a clear energy window to monitor the structure of change relative to C-H bonds. The team obtained the most convincing evidence of C-H bond breaking from Raman maps relative to specific vibrational modes. Alternatively, they could also use a high-speed, single-photon avalanche photodiode (APD) with an edge-tunable band-pass filter to record the TERS maps. They characterized the major difference among the three pentacene species based on the number of C-H bonds in the central ring and during structural transformation. The simulated Raman maps were in good agreement with the experimental outcomes and showed how all vibrational modes maintained highly localized features. For example, the TERS signals were located at the central ring or the outer rings of the species implying highly conjugated pentacene to be partially conjugated. The experimental Raman modes could also be further described by theoretical simulations for the suggested molecular structure. By combining the chemical components obtained using TERS and AFM techniques, the team also verified the possible chemical structures of the γ species.
![Optimized structures and simulated images. (A) Optimized 3D geometries of the pentacene species a,b, and g, with enlarged scale (×5) along the surface normal. (B) Top panel is a side view of the optimized geometries. The slight displacement of Ag atoms along the [001] direction is indicated by the green arrows under b and g. Bottom panel is a side view of the distorted skeletons with enlarged scale (×10) in the surface normal. q1 and q2 denote the distortion of the central benzene ring in a and g, respectively, with respect to the surface plane. d = 0.44 Å, and 0.31 Å labels the displacement of central C atom along the surface normal direction from a to g. (C) Simulated constant current STM images with integrated DOSs in the range from 0.2 to 0.3 V. The images have been processed with Gaussian smoothing with an SD of 1.33 Å. (D and E) Simulated AFM images and electron density maps of a, b, and g. AFM images are simulated with the effective lateral stiffness k = 0.5 N·m−1 and q = 0.2e. Credit: Science, doi:10.1126/science.abd1827 Understanding the structural and chemical heterogeneities of surface species at the single-bond limit](https://scx1.b-cdn.net/csz/news/800a/2021/14-understandin.jpg)
Outlook
The combined experiments using STM, AFM, and TERS (scanning-tunneling microscopy, atomic force microscopy and tip-enhanced Raman spectroscopy) additionally provided a better reference parameter for selection during density functional theory (DFT) simulations. For example, the STM image simulations reproduced the characteristic rod-like, dumbbell-like and spindle-like features for α, β and γ respectively, albeit with marginal error, which Xu et al. clarified relative to reliable structural information. In this way, Jiayu Xu and colleagues showed how modern tip-based techniques could be used to characterize surface chemistry in materials science. Using a joint strategy of STM-AFM-TERS, they experimentally determined the interrelated structure and chemical heterogeneities of surface species relative to these pentacene species on a metallic surface. The experimental protocol detailed in this work can be widely applied to study the surface chemistry and catalysis at the single-bond limit in materials science.
More information: Xu J. et al. Determining structural and chemical heterogeneities of surface species at the single-bond limit, Science, 10.1126/science.abd1827
Zhang R. et al. Chemical mapping of a single molecule by plasmon-enhanced Raman scattering, Nature, doi.org/10.1038/nature12151
Riss A. et al. Imaging single-molecule reaction intermediates stabilized by surface dissipation and entropy, Nature Chemistry, doi.org/10.1038/nchem.2506
Journal information: Science , Nature Chemistry , Nature
© 2021 Science X Network