This article has been reviewed according to Science X's editorial process and policies. Editors have highlighted the following attributes while ensuring the content's credibility:
fact-checked
peer-reviewed publication
trusted source
proofread
New method reveals folding speed limit of helical membrane proteins
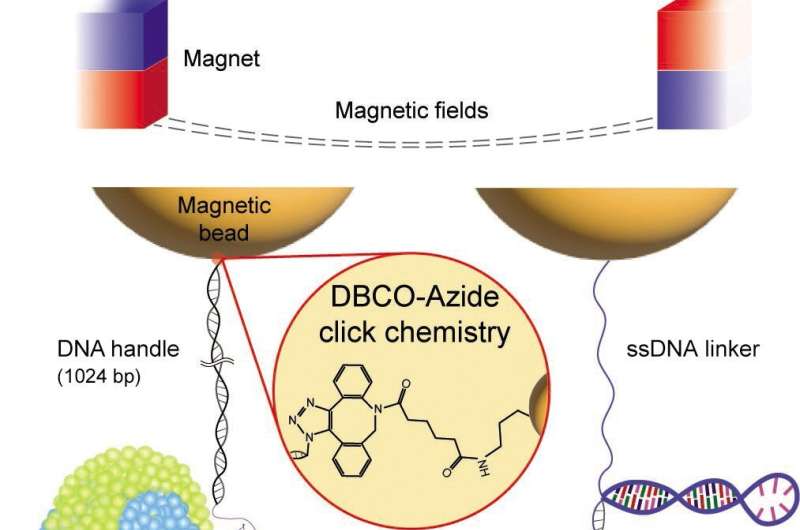
Membrane proteins play a pivotal role in various cellular functions and are key targets for pharmaceutical interventions. In fact, approximately 60% of drugs currently available on the market target these specific proteins. To develop effective drugs that interact with membrane proteins, it is imperative to comprehend their structures and principles underlying their folding processes.
Recognizing this critical need, Professor Duyoung Min and his research team in the Department of Chemistry at UNIST embarked on a study to unravel the folding dynamics of helical membrane proteins.
By developing a robust single-molecule tweezer method using dibenzocyclooctyne cycloaddition and traptavidin binding, the team successfully estimated the folding "speed limit" for these proteins. The findings provide valuable insights into structural states, kinetics, and energy barrier properties critical to advancing our understanding in this field.
Single-molecule tweezers, including magnetic tweezers, have emerged as powerful tools for investigating nm-scale structural changes within individual membrane proteins under force. However, previous studies were limited by weak molecular tethers that hindered long-term observation of repetitive molecular transitions due to force-induced bond breakage. Overcoming this challenge was crucial for obtaining reliable characterizations of structural states and kinetics.
In their study, published in the May 2023 issue of eLife, Professor Min and his research team introduced an innovative single-molecule tweezer method with exceptional stability compared to conventional linkage systems. This new approach demonstrated lifetimes over 100 times longer than existing methods—surviving up to 12 hours at forces as high as 50 pN—and allowing for approximately 1,000 pulling cycle experiments.
Leveraging this advanced technique, the research team observed numerous structural transitions within a designer single-chained transmembrane homodimer for nine continuous hours at constant forces of 12 pN. These observations provided unprecedented insights into its folding pathway and revealed previously hidden dynamics associated with helix-coil transitions.
To accurately characterize energy barrier heights and folding times during these transitions, the researchers employed a model-independent deconvolution method combined with hidden Markov modeling analysis. The results obtained using the Kramers rate framework unveiled a remarkably low-speed limit of 21 milliseconds for helical hairpin formation in lipid bilayers, contrasting with the microsecond scale typically observed in soluble protein folding. This discrepancy can be attributed to the highly viscous nature of lipid membranes, which retards helix-helix interactions.
These findings offer a more valid guideline for understanding the kinetics and free energies associated with membrane protein folding—a critical factor in drug development targeting membrane proteins. As approximately 60% of drugs on the market focus on these proteins, this research opens up new avenues for enhancing pharmaceutical research and design.
More information: Seoyoon Kim et al, Robust membrane protein tweezers reveal the folding speed limit of helical membrane proteins, eLife (2023). DOI: 10.7554/eLife.85882
Journal information: eLife