This article has been reviewed according to Science X's editorial process and policies. Editors have highlighted the following attributes while ensuring the content's credibility:
fact-checked
trusted source
proofread
Searching for dark matter with the world's most sensitive radio
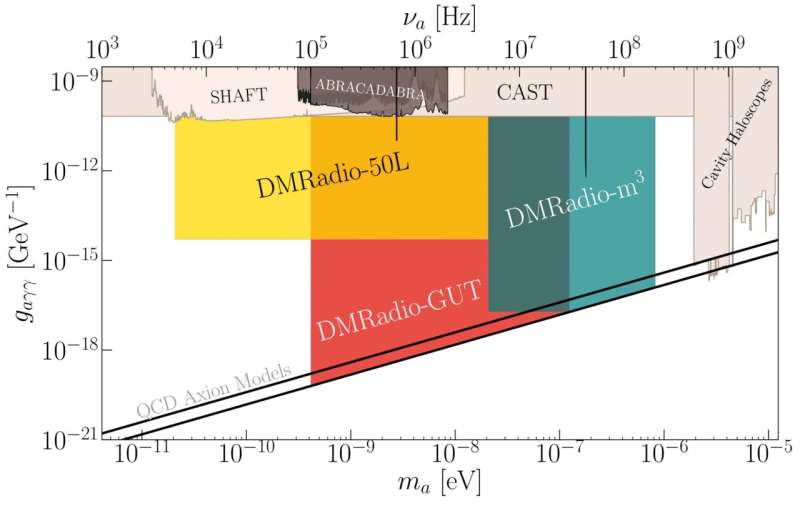
Since the 1960s there has been plenty of evidence to support the existence of dark matter through astrophysical and cosmological observations, and at this point we're very confident that it exists. The question remains, though: what is dark matter actually made of?
Axions: A two-for-one solution?
Throughout the decades there have been many candidates for dark matter, such as weakly interacting massive particles (WIMPs), neutrinos, and primordial black holes. Candidates like WIMPs were originally theorized because they have properties that address issues in other parts of physics. Another candidate that could answer some thorny physics questions is called the axion.
Axions were originally theorized as a solution to a particle physics question known as the Strong CP Problem, but physicists also realized that axions could be produced in a way that would satisfy requirements for them to be dark matter. These are the particles that the DMRadio experiments search for.
Axion detection schemes
When we come up with new theories in particle physics, we try to solve existing problems without making new ones. In the case of axions we solve the Strong CP problem while, for example, still making sure our theories obey special relativity, the standard model, and other established facts in physics. New models in particle physics are expected to interact with our established models in every possible way, as long as those interactions don't break our original theory.
For axions this means that while they may solve the Strong CP Problem they can also do more familiar things that could make it easier for us to detect them; namely, when they come across a magnetic field in space, they may sometimes interact with it to produce an electric field.
When this process occurs, we can actually think of the electric field that the axion is producing as something that looks like a current oscillating at some frequency. Since, of course, the dark matter interacts so weakly with everything we know, this current is expected to be very, very small; approximately 1016 times smaller than what you'd get in a typical lightbulb.
So then, how do we actually try to measure this current?
We make devices that would resonate if this current were inside them—these are devices that look like circuits or metallic cavities. For example, if you hold a guitar string taut with your hands, it will oscillate; but if you then take that string and put it on the body of a guitar, the body will resonate and make that sound much louder. Similarly, we build resonators, which in many ways look like circuits, and these make our (electromagnetic) signal much louder.
DMRadio
In DMRadio we work on three experiments that look for axions at different frequency ranges (with some overlap); these experiments are DMRadio-50L, DMRadio-m3, and DMRadio-GUT. The names of the first two experiments refer to the rough volume of the detector (50 liters and a cubic meter, respectively), while GUT refers to grand unified theories which could be responsible for the types of axions the experiment could detect.
For DMRadio-50L, we use a toroidal magnet to produce the magnetic field (B0 in Figure 2), which implies that axions then produce an oscillating current that flows in a loop (Jeff). At this point, the problem has been reduced to one of classical electrodynamics: How can we detect a very small current oscillating in a circle?
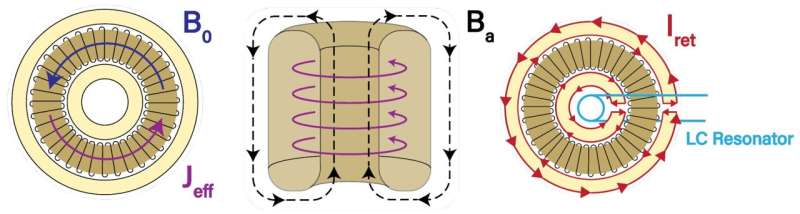
To measure this signal, we first wrap a superconducting sheath around our magnet onto which the axion-induced currents can flow. Then, in the center of our toroidal sheath, we put an inductor which picks up the magnetic flux produced by Jeff. While this setup would in theory pick up our axion signal, we enhance the signal by connecting a tunable capacitor to the inductor; this makes the circuit resonant and tunable. Ultimately sensors like direct current SQUIDs or Radiofrequency Quantum Upconverters, which our own lab produces, pick up the axion signal.
DMRadio-50L is currently being assembled on Stanford's campus in the basement of the Physics and Astrophysics Building where it will start taking data soon. The frequency ranges it will cover are 5 kHz–5 MHz, which correspond to axions with a mass of 20 peV/c2–20 neV/c2; that is, more than 20 trillion times lighter than an electron (these masses correspond to the yellow region in Figure 1). While this will not be sensitive to the most promising axion candidates, known as QCD axions, it will search for axions that have not been probed by any other experiment.
DMRadio-m3, while operating under the same assumptions, has a different geometry (Figure 3). Instead of using a toroidal magnet, we now use a solenoidal magnet where the magnetic field predominantly points vertically inside the magnet—that in turn means that the axion current also points in the same direction. We then build a coaxial pickup on which the magnetic flux from the axion induces currents. We then again tune the resonance of this structure using a capacitor. This experiment, which is being built at SLAC National Lab, will look for higher mass axions than DMRadio-50L, namely axions with masses of 20–830 neV/c2 (the green zone in Figure 1), and it will also have the ability to discover QCD axions.
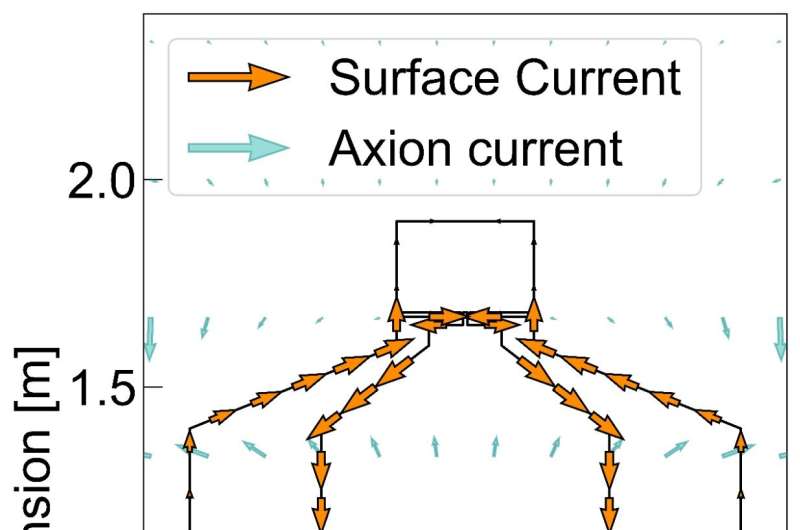
The third experiment, DMRadio-GUT, is a future experiment which is still in the preliminary design phase. We expect to use the experience we gain from the design and operation of DMRadio-50L and DMRadio-m3 to design this experiment, which will ultimately search for QCD axions with a mass of 0.4–120 neV/c2—that is, the masses covered by DMRadio-50L but with significantly higher sensitivity (the red section in Figure 1).
These experiments will enable us to search for axions in places we've never looked before while also allowing us to test new quantum devices. Whether we find the axion and dark matter is of course unknown, but, regardless, we are bound to learn a lot as we design and operate our DMRadios.
Provided by Stanford University