October 2, 2013 feature
The lightness of being: Smaller computer logic components through photon-molecule interaction
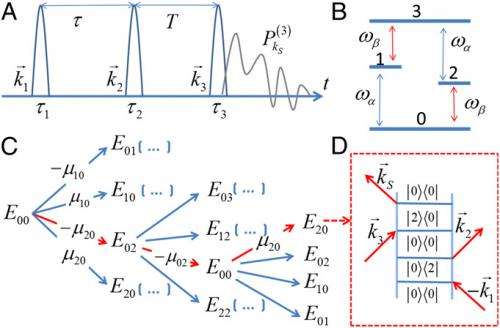
In the unending effort to reduce the size of computing nodes, scientists at University of Liège, Belgium, The Hebrew University, Jerusalem, University of Padova, Italy, and University of California, Los Angeles have employed molecules for the task of evaluating logic functions. Their bilinear process – which detects and measures interactions between light and matter through a synthesis of two-dimensional three-photon echo spectroscopy and Lie algebra – is more effective if multiple operations are performed in parallel, thereby supporting both binary and quaternary logic. Moreover, say the researchers, their simulations enhance classical logic complexity by allowing relaxation by weak coupling to the environment.
Prof. Raphael D. Levine spoke with Phys.org about the paper that he, Prof. Francoise Remacle and Dr. Barbara Fresch of Liege University and their co-authors recently published in Proceedings of the National Academy of Sciences. "Logic deals with propositions," they explained. "It's simpler to deal with binary, so called on-off, propositions." In such cases, an assertion, condition or state either is or is not true. At the same time, there are ternary, quaternary or higher valued logic. "For example," Levine illustrates, 'the balance in your bank account' can take three values: overdrawn, empty or positive.
Another factor, Levine adds, is the need to establish relations between propositions, and so-called bilinear relations – that is, those that involve two propositions by employing logical operators such as AND and OR – are the simplest to work with. "In the 19th century," he notes, "George Boole showed mathematically that AND and OR suffice to express all combinations of propositions, and in 1938 Claude Shannon demonstrated how one can implement both bilinear operations using electrical switches." These insights led to today's computers.
The main obstacle, Levine tells Phys.org, is that while switch size has been reduced to half its value roughly every two years, this trend is reaching its limit as size approaches atomic dimensions. "Our challenge is to perform more logical operations than what is possible with a switch, on a faster time scale, with perhaps multivalued propositions and perhaps multitasking, in which several logical processes are executed in parallel."
Levine and his colleagues addressed this challenge by using light-matter interaction to implement bilinear classical logic. "Light lends itself naturally to make binary propositions because it can be on or off," Levine points out. "However, light's real potential is that it can be switched between on/off states much faster than an electrical voltage can.' More specifically, while the electrical signal used to determine computer clock speed can be switched in a nanosecond, light can be switched up to a million times faster – but at a much higher cost.
"The challenge in implementing bilinear logic through light-matter interaction," Levine acknowledges, "is to build a conceptual bridge between observing such transitions and the evaluation of logic propositions." Matter can exist in many states, Levine continues, so it's very well suited to implementing binary or multivalued logic where each state is a possible answer to the proposition. Moreover, a change in the state of matter is induced by the light being switched on. It is thereby a bilinear relation that depends on both light and matter.
The researchers employed weak-field spectroscopy to achieve nontrivial measurements because a strong laser field results in molecular deformation. In addition, they implemented a molecular decision tree using the bilinear laser system's three pulses in a 2D photon echo spectroscopy experiment to generate a rich parallel logic. "With three laser pulses one can implement operations between three logical variables," Levine explains. "Each laser pulse generates action in parallel because, since the molecule is a quantum mechanical object, it can simultaneously follow several paths." As a result, it can represent all outcomes of an n-dimensional logical operation.
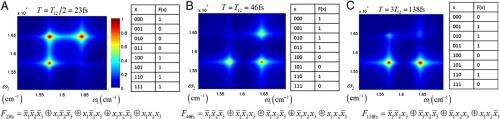
2D-photon echo spectroscopy provides information on dissipation, dephasing, solvation, and electronic or vibrational interstate coupling mechanisms in various multistate material systems, and relies on the measurement of the third-order polarization of the chromophore – the component of a molecule responsible for its color, which arises when a molecule absorbs certain wavelengths of visible light and transmits or reflects others. "In order to create the third-order polarization, the system has to interact three times with a laser pulse," Levine explains. "The state of the system is described quantum mechanically by the density matrix, meaning that we do not describe only the populations of the four accessible states but also coherences between them – for example, the density matrix of a four-level system has 4X4=16 entries. Each interaction with the laser field couples different elements of the density matrix and system state evolution – coupling that can be expressed through a set of possible paths, known as Feynman paths. The main idea here is to map a subset of Feynman paths into the branches of a logic decision tree."
Another factor in their research was that of using Lie algebra – an algebraic structure which studies infinitesimal transformations – to generate the outcome of bilinear light–matter interaction. As with other forms of algebra, a Lie algebra deals with operations on objects, typically binary operations and propositions. What's special about a Lie algebra, Levine explains, is that a binary operation between two objects returns an object, making the outcome more easily measureable.
In addressing these challenges, Levine says, the essential innovation of their paper is bringing together very diverse techniques to bear on the problem of logic on the molecular scale. "We're able to do that largely because we've been honing our approach for about a dozen years. Our first papers, from 2000-2001, were somewhat ahead of their time – but," he quips, "now the world is catching up with us."
Levine points out that their paper is a joint theoretical-experimental effort (with the experiment being conducted in Prof. Elisabetta Collini's lab). "In the paper and its supplement, we only talk about what can actually be implemented in the laboratory," he emphasizes. "This may seem obvious, but actually it's not – we could develop very elegant schemes that suffer only one drawback: They can't be realized with currently available technology." In terms of theory, he adds, the scientists integrate multiphoton laser quantum spectroscopy (including the ability to numerically simulate the output of the experiments), the Lie algebraic approach to doing dynamics, and the notion of a decision tree. "Such synergism," Levine stresses, "is not so easy to actually achieve."
Levine defends deciding to address binary rather than quaternary logic in their paper as "a purely pragmatic decision, since for the typical scientist binary is conceptually much easier than quaternary – and current logic devices are binary because of the long-standing supremacy of transistors as logic building blocks. However," he adds, "in terms of efficiency per operation, quaternary is twice as powerful as binary, and four times as powerful as binary per two operations, eight times as powerful as binary per three operations, and so on." (This relationship varies according to a function of 2n, where n is the number of parallel operations.) "That's why our real aim is to do quaternary logic – because the power law would scale as a function of 4n."
The study's simulations allow relaxation by weak coupling to the environment, which adds to the complexity of the logic operations. "The experiment is done with the molecule in solution, usually water," Levine explains. "The interaction with the polar water molecules can induce changes in the molecule not unlike the changes induced by the lasers, which opens up new alternatives. Having different alternatives is the name of the game."
Levine also points out that their findings can be scaled up either by increasing the number of interactions or, more simply, by increasing the number of accessible transitions. "Every interaction is equivalent to another level in a decision tree, meaning that we include another logical variable in the function to evaluate. Again, more interactions allows for a more complex logic, in the sense that a function with a larger number of logic variables can be evaluated." Basically, the number of accessible transitions is the number of possible answers to a question. "For example," Levine illustrates, "quaternary logic needs four different transitions, so the application of our scheme to more complex chromophores, for which more transitions are possible, allows the implementation of multivalued logic."
Along similar lines, the binary implementation described in the paper can be generalized to multivalued logic, Levine notes, because there are no conceptual limitations in extending their formalism to multivalued logic. "What we need is a system able to undergo several transitions, and nature offers a variety of such systems – for instance, light harvesting systems in photosynthetic organisms are usually composed of several chromophores. However," he points out, "there are practical limitations to consider: In the readout process one needs to distinguish as many values as the elements of the multivalued logic one wants to use. This means that to do binary logic one needs to be able to reliably distinguish between two possibilities, to do ternary logic one needs to distinguish three possibilities, and to quaternary one needs to distinguish four possibilities. In other words, the maximum radix of the logic is limited by the experimental resolution one can achieve in the experiment with a given system. Moreover, one also needs to make the distinction promptly. This is a pragmatic question and has to do with the limitations imposed by technology."
In terms of next steps, Levine tells Phys.org, the researchers might take advantage of other properties of light-matter interaction to obtain fast and reliable logic operations. "For example," he illustrates, "we do not yet use the fact that the polarization of each laser light can be controlled, allowing further selectivity on the accessible transitions contributing to the final spectra."
There's also a major challenge that the scientists know how to address electronically but not optically – specifically, using wires to deliver an electrical pulse to a single switch. Therefore, while they can employ integrated circuits in the former case, they need to determine how to usefully deliver an optical pulse to a single molecule – and the experiments they describe involve very many molecules each acting independently. "Another major challenge effectively addressed by electrically," Levine continues, "is the cascade of information from one computing unit to the next. Electrically, one can amplify the signal and have gain, but proposing a scheme for efficiently cascading information through several optically addressed computing units is a crucial research direction."
In addition, notes Remacle, the current research is part of the collaborative MULTI Unconventional Computing project (funded by the European Community FET proactive program of FP7) focused on implementing complex logic in molecular systems. MULTI refers to the extension of current logical paradigms to multivalued logic implemented in multistate systems leading to multitasking molecular logic processing. "The next steps in this direction will be developing the multivalued capabilities of our approach, along with the demonstration that the concepts developed in this paper can be transferred to other experimental setups, such as electrically addressed molecules."
Levine identifies other areas of research that might benefit from their study. "Molecular logic is intrinsically an interdisciplinary field," Levine notes. "If molecules are useful to implement complex logic operations, it's also true that the description of physico-chemical processes in terms of logic offers a new perspective on the physics itself. The exploitation of this different perspective," he concludes, "can be useful in a variety of research areas from material science and nanosensors to molecular biology and nanomedicine."
More information: Molecular decision trees realized by ultrafast electronic spectroscopy, PNAS Published online before print September 16, 2013, doi:10.1073/pnas.1314978110
Journal information: Proceedings of the National Academy of Sciences
© 2013 Phys.org. All rights reserved.