October 21, 2019 feature
The DUNE experiment could lead to new discoveries about solar neutrinos
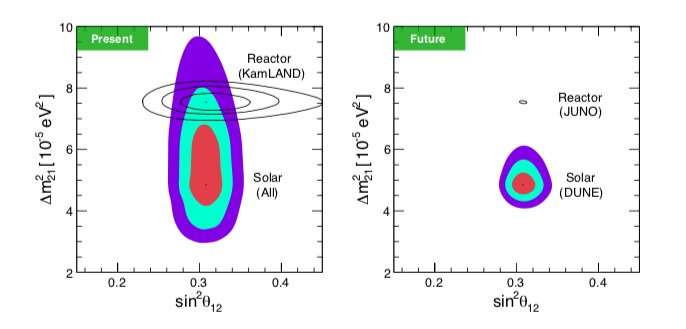
The Deep Underground Neutrino Experiment (DUNE) is an international research collaboration aimed at exploring topics related to neutrinos and proton decay, which should start collecting data around 2025. In a recent study featured in Physical Review Letters, a team of researchers at Ohio State University have showed that DUNE has the potential to deliver groundbreaking results and insight about solar neutrinos.
Neutrino astronomy is a field that studies the different types of neutrinos. Research in this field, such as the recent study carried out by the team at Ohio State University, has increased dramatically over the past few decades.
"As far as we know, neutrinos are elementary particles, meaning that they are not composed by 'smaller pieces,'" Francesco Capozzi, one of the researchers who carried out the study, told Phys.org. "These particles do not possess any electric charge so they cannot interact electromagnetically via the force that keeps electrons and protons together in an atom."
Neutrinos are fascinating particles, as their main properties are very different from those of other elementary particles. For instance, their masses are incredibly small, approximately one-millionth of the next-lightest particles (i.e., electrons).
Another unique characteristic of neutrinos is that they can only interact with other matter via a so-called 'weak interaction.' As suggested by its name, this 'weak interaction' is much weaker than the electromagnetic one; so weak that neutrinos can travel through the Earth or sun without ever interacting with other particles. In addition, as they are neutral in charge, neutrinos are not affected by the magnetic fields of the Earth or sun.
"The properties of neutrinos make them unique probes of the universe," Capozzi said. "They can carry information of regions which would be inaccessible otherwise."
There are several sources of neutrinos, and depending on where they are produced, they can differ in fluxes, energies per particle, and other properties. Solar neutrinos, for instance, are produced in the sun's core, but can then escape to other parts of the solar system. Approximately 60 billion electron neutrinos per square centimeter reach the Earth from the sun every second. Analyzing these particles could allow researchers to uncover real-time information about what is happening at the very center of the sun.
Another type of neutrino comprises those produced during the super energetic explosions of massive stars, supernova neutrinos. These neutrinos reach Earth hours before the light produced in the explosion, and come directly from the innermost part of an exploding star, where the density is so high that even neutrinos can be trapped for a while. These are just a few examples of neutrino sources, but there are countless others, some of which have not yet been detected.
"There even exist neutrinos that have been propagating freely through the universe since about one second after the Big Bang, which carry the imprints of the primordial universe," Capozzi said. "However, we have still been unable to detect these."
Based on what astrophysicists have observed so far, neutrinos come in three main 'flavors': electron neutrinos, muon neutrinos and tau neutrinos. Each of these different 'flavors' is identified based on the charged particle produced during a weak interaction (i.e., electrons, muons or taus).
So far, detecting and studying neutrinos has proved incredibly challenging, mainly due to the fact that they rarely interact with other matter. One way to overcome this limitation is by building large detectors that compensate for the low probability of neutrino interactions by increasing the number of possible particles they can interact with.
The Super-Kamiokande (Super-K) detector in Japan, which essentially consists of a tank filled with 50,000 tons of the purest water available on Earth, is currently the largest detector available for MeV (low-energy) neutrinos. Low-energy neutrinos are those in the MeV energy range, which are mainly produced in nuclear processes, for instance, via fusion reactions in the sun or at the center of exploding stars.
"Another problem is that we cannot see neutrinos themselves using detectors; we can only see the charged particle produced in their interactions," Capozzi explained. In Super-Kamiokande, for instance, we see the light these charged particles emit in water when traveling at almost the speed of light."
The sun is one of the most important natural sources of neutrinos, as they are produced via the same nuclear reactions that allow the sun to shine. When scientists first started detecting solar neutrinos in the 1960s, they found that there were fewer electron neutrinos than they expected.
"A possible explanation for this anomaly was that neutrinos were changing their flavor while propagating," Capozzi explained. "This phenomenon, now known as neutrino oscillation, is physically possible only if neutrinos have mass. It took about 30 years to confirm that the solar neutrino anomaly was, indeed, due to neutrino oscillations."
Essentially, although neutrinos come in different flavors, scientists discovered that they can also oscillate and 'change flavors.' The two physicists who discovered this, Takaaki Kajita and Arthur B. McDonald, were awarded the Nobel Prize in Physics in 2015.
"Perhaps the weirdest thing about neutrinos is that they oscillate," Shirley Li, another researcher involved in the study, told Phys.org. "Neutrinos born with one flavor may turn into neutrinos with a different flavor after they propagate over some distance. Imagine how surprised you would be if you bought a cup of chocolate ice cream and see that it turned into strawberry ice cream once you open it at home. Physicists were equally surprised when neutrino oscillation was discovered."
Since the discovery of neutrino oscillations, researchers have been using solar neutrinos to determine the parameters describing their oscillations. Despite the huge efforts to achieve this, many questions remain unanswered.
Firstly, researchers were unable to observe all of the nuclear reactions through the correspondent neutrinos. For instance, 'hep' neutrinos, which are produced from the fusion of a nucleus of Helium and a proton, have proven particularly difficult to observe. In fact, while hep neutrinos are the most energetic among solar neutrinos, they are very small in flux compared to other neutrinos.
In addition, the oscillation parameters determined in solar neutrino experiments are not in complete agreement with the measurements collected in other types of experiments. This could be due to some unknown physical phenomena that only affect solar neutrinos.
"We have not run out of questions about solar neutrinos, we have run out of detector advances," John Beacom, one of the researchers who carried out the study, told Phys.org.
Due to the limitations of existing detectors, most current neutrino experiments will most likely be unable to address unanswered questions. This inspired a large and international team of researchers to start building DUNE, a large detector in a mine in South Dakota that is 4850 feet underground.
"In the past, it has already been discussed that DUNE might be used as a solar neutrino detector, too," Capozzi said. "However, no thorough study in this direction has been performed. We decided to cover this gap, showing that DUNE is actually able to provide the answers to those questions, with basically no extra money investment."
In their recent study, Capozzi, Li, Beacom and their colleague Guanying Zhu set out to prove that the DUNE mine could also be a world-leading solar neutrino detector. In order to do this, they first assessed the mine's amount of background, which is essentially something observed in a detector that mimics the signal that one is looking for, even if it has an entirely different origin. This background can confuse and adversely affect the measurement and detection of neutrinos.
"In the energy range relevant for solar neutrinos, the most important background is coming from natural radioactivity," Capozzi explained. "Since the experiment will be based in a cave in a deep mine, the radioactivity comes from the surrounding rock. In order to make an estimate of the background, we first have to understand the rock composition expected for the detector site."
Simulating background events in DUNE proved somewhat challenging, as these can come from a variety of sources, and thus to identify them requires in-depth analyses. When they first started working on their study, the researchers thus started investigating the background sources for neutrino experiments carried out in the past and computed these rates in the context of DUNE.
"It turns out that their rates are reasonably low compared to the signal rates," Li said. "However, halfway through our study, we discovered in the literature the existence of this particular background only for argon detectors. These are low-energy neutrons produced from radioactivities in surrounding rock. This turns out to be the dominant background for solar neutrino measurement in DUNE."
The researchers based their analyses on past literature that outlines the geological aspects of DUNE's mine, which are of crucial importance to complete proper excavations. Knowing the exact rock composition in the mine, they were then able to carry out a calculation to predict its expected background. Subsequently, they used statistical tools to evaluate the precision that DUNE can reach in measuring the oscillation parameters and the flux of neutrinos escaping from the sun.
Once they identified the possible sources of background in the DUNE experiment, they tried to come up with strategies to eliminate the backgrounds, as their rates are typically much higher than the neutrino signal rates. They came up with two distinct solutions: one that entails surrounding the detector with a layer of plastic and the other collecting data for twice as long to achieve better sensitivity.
"For each step of the experiment, we had to take care of further details," Capozzi said. "For example, we had to treat the neutrino interactions carefully with the detector, which will be made of liquid argon. At the energy relevant for solar neutrinos, a very important interaction is with the entire argon nucleus, which depends on complicated nuclear effects."
Before they set out to assess the potential of DUNE as a detector to discover new things about neutrinos, the researchers reviewed all past research on this topic, comparing results that were obtained using different experimental and theoretical nuclear physics techniques. Ultimately, they selected the technique that they felt was more appropriate and implemented it using local computers at their university.
"We now have a theoretical framework that allows us to compute the probability of neutrinos born with one flavor tuning into a different flavor," Li said. "This depends on the energy of the neutrino and the propagation distance, as well as six oscillation parameters. We wanted to measure as many types of neutrino oscillation as we could, e.g., neutrinos with any of the three flavors oscillating to other flavors, in order to get the most precise measurements of the six oscillation parameters, and most importantly, to evaluate whether our current framework of neutrino oscillation was, indeed, correct."
The DUNE experiment is designed to study neutrinos specifically by measuring a flux of high-energy muon neutrinos oscillating to electron neutrinos reaching a big detector the South Dakota-based underground mine. This could ultimately allow researchers to measure two oscillation parameters that were roughly measured in previous experiments with greater precision.
The detector used in the DUNE experiment is extremely large compared to other existing detectors. It is 40 kilotons of argon with which neutrinos can interact, and it detects particles via a time projection chamber technology, enabling the collection of 3-D images for each neutrino interaction.
"A natural question is, what else can this amazing detector measure?" Li said. "This is how we got the idea to measure solar neutrinos with DUNE. Solar neutrino oscillation is particularly interesting. So far, solar neutrino and reactor neutrino oscillation patterns slightly disagree. This could due to two reasons: Either there is an unlikely statistical fluctuation in the current data, or our current theoretical understanding of neutrino oscillation is not complete. The second possibility is extremely exciting."
Another experiment called JUNO, planned for 2020, will measure neutrinos coming out of nuclear reactors. Solar neutrinos and reactor neutrinos are, in principle, sensitive to the same oscillation parameters. So if the theoretical framework proposed by the researchers is right, the parameters identified in the JUNO experiment (i.e., for reactor neutrinos) should be aligned with those gathered in the DUNE experiment (i.e., for solar neutrinos).
Past studies have found discrepancies between the oscillation parameters of reactor and solar neutrinos, but the more precise measurements that are set to be collected in the JUNO and DUNE experiments could shed some light on this discrepancy. This could in turn lead to the discovery of new physical phenomena.
So far, there has been some skepticism in the physics world about the DUNE underground mine being an effective solar neutrino detector, primarily due to the large amount of background expected, which could adversely affect results. In their study, however, Capozzi, Li, Zhu and Beacom demonstrated that DUNE could lead to world-leading measurements of solar neutrinos, while also potentially enabling the first-ever precise measurements of 'hep' solar neutrinos.
"Despite the skepticism, we were able to show that this background can be drastically reduced applying some selection criteria to what we will see in the detector," Capozzi said. "The background left will only dominate over the signal at low energies. The high-energy part will be 'untouched,' and we estimated that it will consist of 100,000 solar neutrinos observed in five years."
In addition to demonstrating the huge potential of DUNE, Capozzi Li, Zhu, and Beacom introduced a series of feasible theoretical and experimental improvements that could enhance the performance of the DUNE detector. These improvements could also benefit the project at large, facilitating the investigation of other physical phenomena.
In order to address unanswered questions, the next generation of neutrino detectors will need to be huge in size and have advanced detection capabilities. Even using these detectors, some of the properties and characteristics of neutrinos will most likely remain a mystery, as there are still numerous technical challenges to overcome.
"Without this measurement in DUNE, we may never know why solar neutrinos appear to mix differently from reactor antineutrinos," Beacom said. "We are not saying that investigating this will be easy, but we are saying that it is important."
The researchers at Ohio State University are now planning to share the results of their calculations and simulations, as well as their suggestions for improvement with the astrophysics community at large. They hope that this will spark conversation and ultimately encourage changes that could further enhance the performance of the DUNE detector before the experiment is carried out.
"We are excited to see that the DUNE collaboration is looking into the details of our analysis, and hopefully we will see this analysis carried out when DUNE comes online," Li said. "Overall, it is a truly exciting time to study neutrinos, as there are so many interesting measurements and tests one can do in these experiments. I am still trying to come up with measurements that haven't been considered before and study what they can tell us about neutrinos and physics beyond the Standard Model."
One of the core goals of the team's future research will be making the most out of the observations gathered in the DUNE mine or using other large detectors. To do this, the team plans to continue investigating new techniques that could make detectors more sensitive to neutrinos produced from astrophysical sources.
More information: Francesco Capozzi et al. DUNE as the Next-Generation Solar Neutrino Experiment, Physical Review Letters (2019). DOI: 10.1103/PhysRevLett.123.131803
Y. Fukuda et al. Evidence for Oscillation of Atmospheric Neutrinos, Physical Review Letters (2002). DOI: 10.1103/PhysRevLett.81.1562
Q. R. Ahmad et al. Direct Evidence for Neutrino Flavor Transformation from Neutral-Current Interactions in the Sudbury Neutrino Observatory, Physical Review Letters (2002). DOI: 10.1103/PhysRevLett.89.011301
Journal information: Physical Review Letters
© 2019 Science X Network