January 25, 2021 feature
Terbium (III)-doped fluorescent glass for biomedical research
![Tb3+-doped pipettes emit green fluorescence. (A) Partial energy level diagram of Tb3+ [extracted partially from (14)]. (B) Excitation (blue) and emission (green) spectra of 3.1 mol % Tb3+-doped glass. (C) Macroscopic color photograph of control (top) and Tb3+-doped (bottom) glass capillaries. Photo credit: Yuji Ikegaya, The University of Tokyo. (D) Bright-field monochromatic (top) and fluorescence (bottom) images of the tips of control (left) and Tb3+-doped (right) glass pipettes (488-nm laser excitation at 25 mW). Pipettes made of Tb3+-doped glass emitted green fluorescence. Photo credit: Kazuki Okamoto, The University of Tokyo and Juntendo University. (E) Scanning electron microscopy image of the tip of a Tb3+-doped pipette. Photo credit: Hiroyuki Hioki, Juntendo University. (F) Pipette resistances of control (black) and Tb3+-doped pipettes (green). The rectangles show the medians and the 25th and 75th percentiles, and the whiskers display the 10th and 90th percentiles. n = 48 pipettes, Student’s t test. (G) The same as (F) but for pipette capacitances. n = 7 to 8 pipettes, Student’s t test. Credit: Science Advances, doi:10.1126/sciadv.abd2529 Terbium (III)-doped fluorescent glass for biomedical research](https://scx1.b-cdn.net/csz/news/800a/2021/terbiumiiido.jpg)
Optical investigations and manipulations often form the core of biological experiments. In a new report now published in Science Advances, Kazuki Okamato and a team of scientists in pharmaceutical sciences, neuroscience, medicine, physics and artificial intelligence at the University of Tokyo, Japan, introduced a new borosilicate glass material containing a rare-earth ion terbium (III) (Tb3+). The material emitted green fluorescence upon blue light excitation, much like green fluorescent protein (GFP) with wide compatibility across biological research environments. Using micropipettes made of terbium-doped glass, Okamato et al. targeted GFP-labeled cells for single-cell electroporation, single-cell transcriptome analysis and patch-clamp recording experiments under real-time fluorescence microscopic control. The glass also showed potential third harmonic generation upon infrared laser excitation, useful for online optical targeting of fluorescently labeled neurons in the neocortex in vivo. In this way, the terbium-doped glass simplified multiple procedures in biological experiments with broader applications in biomedical research.
Conducting optical investigations in vivo
Optical investigations and cell manipulations within living tissues are widespread in biological research with capacity to reveal diverse properties in cells and during intracellular communication. While genetic labeling has enabled the identification of cells expressing fluorescent proteins it remains difficult to access fluorescently labeled cells using glass pipettes since glass is not fluorescent in the visible range. To solve these technical problems, Okamato et al. introduced a new composition of borosilicate glass. The rare-earth ions displayed unique fluorescence emission with sharp peaks in the visible light spectrum. The team focused on terbium (III) (Tb3+), which have complex energy level structures and are theoretically expected to emit green fluorescence. The excitation wavelength was near the highest visibility for human eyes and close to that of green fluorescent protein. As a result, pipettes made of terbium-doped glasses were useful for fluorescence-targeted, single-cell manipulation in life sciences.
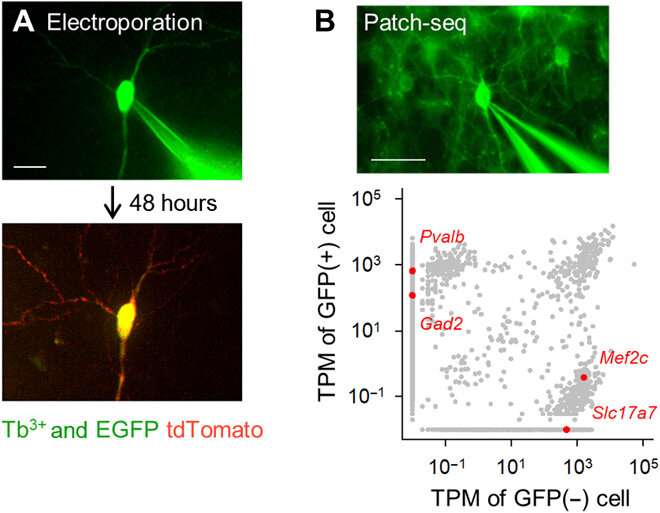
Developing the terbium-doped glass and conducting experiments.
The team developed the borosilicate glass using 3.1 mole percent (mol %) terbium oxide (Tb2O3). The terbium (Tb3+)-doped glass emitted green fluorescence visible to the naked eye, even under room light. The Tb3+-doped glass had an absorption peak at a wavelength of 484 nm, which was not observed in normal borosilicate glass without terbium. The scientists produced glass capillaries and pipettes where the molded glass continued to emit green fluorescence. Using scanning electron microscopy, the team checked the pipette tips for any aberrations that could affect the quality of electrophysiological recordings. Okamato et al. next used the new micropipettes to conduct single-cell electroporation of a red fluorescent protein (tdTomato) into neurons in rat organotypic hippocampal slice structures. They viewed the pipettes containing the red dye vector and pyramidal cells that were minimally labeled with enhanced GFP, using the same optical apparatus. The scientists attached the pipette tips to the cells and applied electrical pulses for electroporation to facilitate the expression of the red dye after 48 hours. Thereafter, the team performed single-cell RNA sequencing with the pipettes in acute slices of the mouse primary motor cortex of transgenic mice.
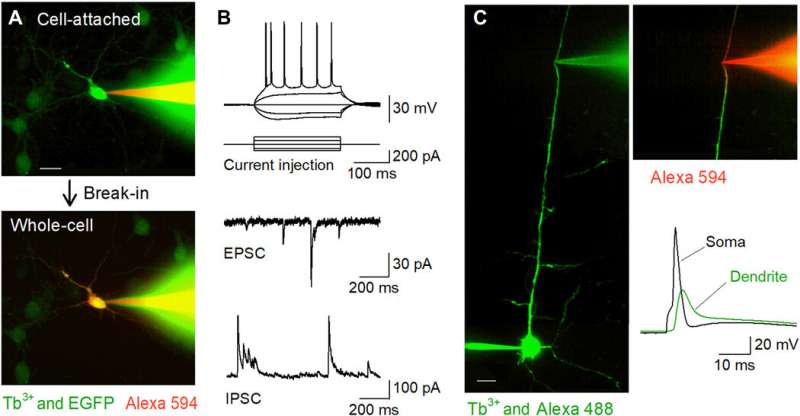
Using the new micropipettes for in vitro patch clamp recordings
The researchers next conducted patch-clamp recordings from primary cultures of hippocampal neurons that were sparsely labeled with GFP using a terbium-doped pipette loaded with a red fluorescent dye (Alexa Fluor 594). The pipettes successfully filled the target cells with the dye and held them in the whole-cell configuration. The team applied the same method to acute brain slice preparations where neurons were located deeper in less transparent tissues than cultured neurons. When Okamato et al. patched pyramidal cells in the acute hippocampal slices using terbium-doped pipettes, the neurons showed normal action potentials in response to brief current injections. The cells exhibited normal spontaneous excitatory and inhibitory post-synaptic currents under the voltage-clamp configuration. The micropipettes could be used for long-term stable recordings and were also useful for recordings from neurites. The team recorded backpropagating action potentials using the setup from targeted dendrites.
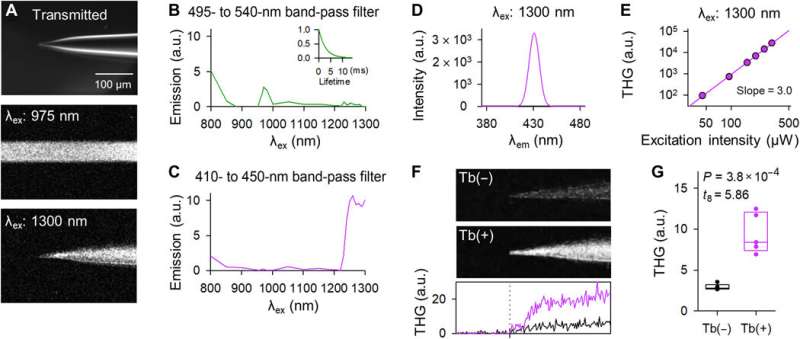
In vivo patch-clamp recordings using the terbium-doped pipettes
Okamato et al. next characterized the nonlinear multiphoton excitation of terbium-doped glass using infrared light at wavelengths that were approximately double the single-photon excitation peak (484 nm) of terbium. Using a photomultiplier tube through a 495-to-540 nm band-pass filter, the team captured the green fluorescence emitted by terbium-doped pipettes. The emissions peaked at an excitation wavelength of 975 nm, suggesting that a laser wavelength corresponding to the value excited the glass through a two-photon absorption process. The scientists also noted another bright signal at 1300 nm excitation through a 410-to-450 band pass filter and suggested the signal to have likely arisen from third harmonic generation (THG). Based on the strong THG signal of the micropipettes, Okamato et al. conducted in vivo whole cell patch-clamp recordings with a multiphoton laser microscope. They simultaneously used the cells and terbium-doped pipette using dual-laser irradiation at 1,040 nm and 1,300 nm, respectively, and recorded the injection-induced action potentials and spontaneous membrane fluctuations under the current-clamp configuration.
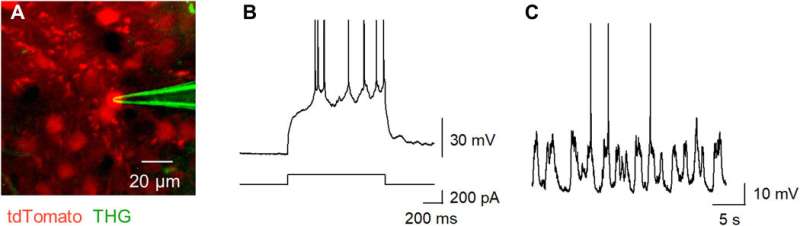
Outlook for biomedical research
In this way, Kazuki Okamato and colleagues invented a terbium-doped glass emitting green fluorescence signal strong enough to be visible to the naked eye. The material had similar characteristics to conventional borosilicate glass and did not display photobleaching or cytotoxicity. The new micropipettes allowed fluorescence manipulations such as optical targeting of single-cell electroporation, single-cell RNA sequencing and electrophysiological recordings. The glass also emitted third harmonic generation upon three-photon excitation, applicable for in vivo manipulation. The terbium-doped glass therefore provided a platform for multiple purposes in biomedical research including hitherto conventional patch-clamp recordings to open new frontiers in life sciences.
More information: Okamato K. et al. Tb3+ doped fluorescent glass for biology, Science Advances, 10.1126/sciadv.abd2529
Chalfie M. et al. Green fluorescent protein as a marker for gene expression. Science, 10.1126/science.8303295
Kitamura K. et al. Targeted patch-clamp recordings and single-cell electroporation of unlabeled neurons in vivo. Nature Methods, doi.org/10.1038/nmeth1150
Journal information: Science Advances , Science , Nature Methods
© 2021 Science X Network