Extracting order from a quantum measurement finally shown experimentally
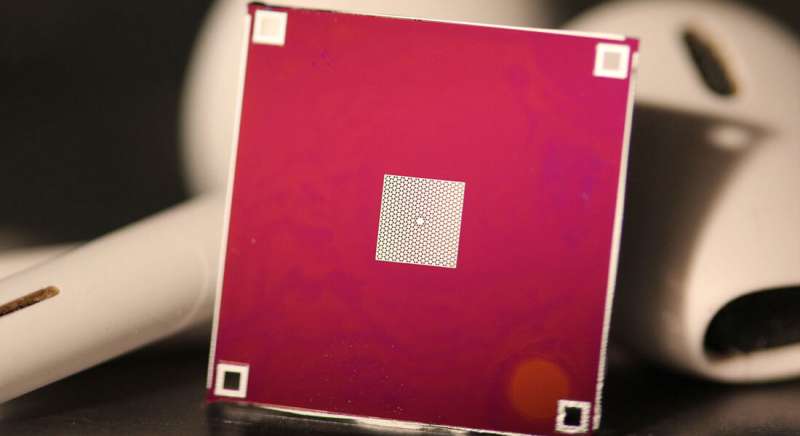
In physics, it is essential to be able to show a theoretical assumption in actual, physical experiments. For more than a hundred years, physicists have been aware of the link between the concepts of disorder in a system, and information obtained by measurement. However, a clean experimental assessment of this link in common monitored systems, that is systems which are continuously measured over time, was missing so far.
But now, using a "quantum drum," a vibrating, mechanical membrane, researchers at the Niels Bohr Institute, University of Copenhagen, have realized an experimental setup that shows the physical interplay between the disorder and the outcomes of a measurement. Most importantly, these outcomes allow to extract order from the largely disordered system, providing a general tool to engineer the state of the system, essential for future quantum technologies, like quantum computers. The result is now published in as an Editors' Suggestion in Physical Review Letters.
Measurements will always introduce a level of disturbance of any system it measures. In the ordinary, physical world, this is usually not relevant, because it is perfectly possible for us to measure, say, the length of a table without noticing that disturbance. But on the quantum scale, like the movements of the membranes used in the Schliesser lab at the Niels Bohr Institute, the consequences of the disturbance made by measurements are huge. These large disturbances increase the entropy, or disorder, of the underlying system, and apparently preclude to extract any order from the measurement. But before explaining how the recent experiment realized this, the concepts of entropy and thermodynamics need a few words.
Breaking an egg is thermodynamics
The law of thermodynamics covers extremely complicated processes. The classic example is that if an egg falls off of a table, it breaks on the floor. In the collision, heat is produced—among many other physical processes—and if you imagine you could control all of these complicated processes, there is nothing in the physical laws that say you can't reverse the process. In other words, the egg could actually assemble itself and fly up to the table surface again, if we could control the behavior of every single atom, and reverse the process. It is theoretically possible.
You can also think of an egg as an ordered system, and if it breaks, it becomes extremely disordered. Physicists say that the entropy, the amount of disorder, has increased. The laws of thermodynamics tell us that the disorder will in fact always increase, not the other way round: So eggs do not generally jump off floors, assemble and land on tables in the real world.
Correct quantum system readouts are essential—and notoriously difficult to obtain
If we turn to quantum mechanics, the world looks rather different, and yet the same. If we continuously measure the displacement of a mechanical, moving system like the "membrane-drum" (illustration 1) with a precision only limited by the quantum laws, this measurement disturbs the movement profoundly. So you will end up measuring a displacement which is disturbed during the measurement process itself, and the readout of the original displacement will be spoiled—unless you can measure the introduced disorder as well.
In this case, you can use the information about the disorder to reduce the entropy produced by the measurement and generate order from it—comparable to controlling the disorder in the shattered egg-system. But this time we have the information on the displacement as well, so we have learnt something about the entire system along the way, and, crucially, we have access to the original vibration of the membrane, i.e. the correct readout.
A generalized framework for understanding entropy in quantum systems
"The connection between thermodynamics and quantum measurements has been known for more than a century. However, an experimental assessment of this link was missing so far, in the context of continuous measurements. That is exactly what we have done with this experiment. It is absolutely essential that we understand how measurements produce entropy and disorder in quantum systems, and how we use it in order to have control over the readouts we shall have in the future from, say, a quantum system like a quantum computer.
If we are not able to control the disturbances, we basically won't be able to understand the readouts—and the quantum computer readouts will be illegible, and useless, of course," says Massimiliano Rossi, Ph.D. student and first author on the scientific article. "This framework is important in order to create a generalized basic foundation for our understanding of entropy producing systems on the quantum scale. That's basically where this study fits into the grander scale of things in physics."
More information: Massimiliano Rossi et al. Experimental Assessment of Entropy Production in a Continuously Measured Mechanical Resonator, Physical Review Letters (2020). DOI: 10.1103/PhysRevLett.125.080601
Journal information: Physical Review Letters
Provided by Niels Bohr Institute