Researcher tackles long-standing mysteries about membrane protein structure
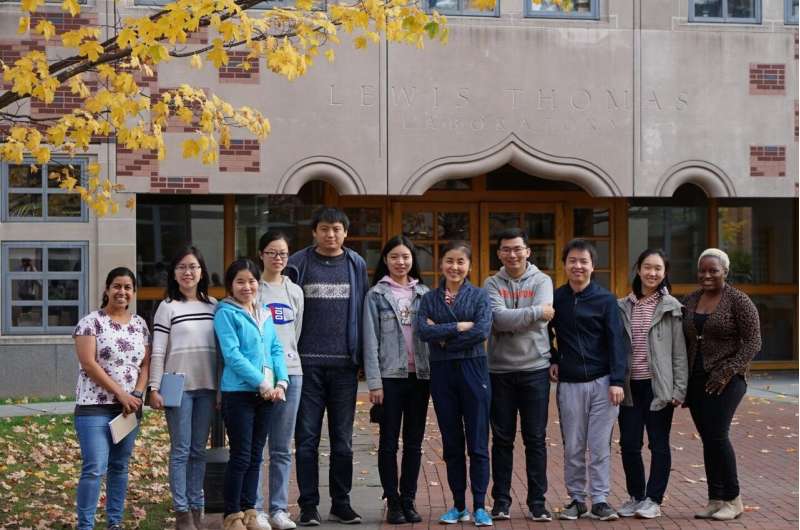
Ion channels and membrane transporters are in the business of moving ions and small molecules across cellular membranes. They are essential for metabolic and cellular homeostasis, and for a host of biological signaling pathways.
"Both these classes of membrane proteins are extremely important for our health," observes Nieng Yan, the Shirley M. Tilghman Professor at Princeton's Department of Molecular Biology. "Defects in these proteins are also associated with many different diseases."
To understand why a defect in a particular protein causes disease, it's important to know not only what that protein does but how it executes this function. Studying a protein's structure lets scientists get a better handle on its mechanisms of action, but the structures of ion channels and membrane transporters have long been shrouded in mystery. That's because technical limitations prevented these proteins from being imaged at high resolutions using existing techniques. However, recent improvements in cryogenic electron microscopy (cryo-EM) have enabled researchers, including Yan, to begin solving this problem for various membrane proteins.
The genesis of Yan's research program has its roots at Princeton, where she was a graduate student in Yigong Shi's laboratory.
"I went to a symposium where the keynote speakers presented this fascinating sterol regulatory element binding protein, or SREBP, pathway. It's the central hub for controlling cellular homeostasis of lipids, cholesterol and fatty acids," says Yan.
The story caught Yan's imagination, and when it came time to choose a graduate research project, she decided ("somewhat naively," she says) to study the complex of SREBP with its escort partner, the ER membrane protein SCAP. SCAP contains a conserved region called a sterol-sensing domain, or SSD, which binds to cholesterol and other sterols.
"Even now," Yan laughs, "we haven't solved the structure of my very first project. Nevertheless, that marked the beginning of my obsession with this whole pathway. Because that project was so difficult, I began to extend my research program to other SSD domain-containing proteins."
Her quest is yielding valuable rewards, with a study published in 2019 on the structure of the SSD-containing human receptor Patched1 in complex with its inhibitor, Sonic Hedgehog. More recently, Yan's group has produced a pair of studies on proteins involved in the development of Niemann-Pick disease type C (NPC): NPC1 and NPC2. NPC1 is a membrane protein found in late endosomes and lysosomes that functions to transport cholesterol across the membrane. NCP2, a small protein that resides inside endosomes and late lysosomes, supplies cholesterol to NPC1.
"Defects in cholesterol transport can cause devastating disease," notes Yan.
Interestingly, NPC1 is also the cellular receptor for Ebola virus. After being endocytosed by a cell, the virus uses NPC1 to escape the endocytic pathway so that it can replicate in the cell's cytoplasm. In a study published in 2016 in the journal Cell, Yan's lab used cryo-EM to obtain the structure of NPC1, as well as a reconstruction of NPC1 in complex with an Ebola surface glycoprotein.
Following on that work is another study in Cell, which appeared in June 2020, exploring the interaction between NPC1 and NPC2. This paper presents a higher-resolution structure of NPC1 alone, and a structure of the NPC1-NPC2 complex. It also describes a pH-sensitive conformational change within NPC1 that may be needed to deliver cholesterol across the membrane. Shape changes are thought to be central to the operation of membrane transporters, but how most proteins change shape to execute their function is still unknown.
"Now our goal is to produce 3-D movies," says Yan, "to capture different conformations of the same molecular machinery to reveal their working cycle."
Ion channels, too, change shape to gate the traffic of charged atoms through the channel; in many channels, this is spurred by a change in voltage across a membrane. Such voltage-gated channels have been a longstanding interest of Yan's. At her first lab at Tsinghua University in China, Yan and her students solved the structure of a voltage-gated human sodium channel, Nav1.7.
"Many animals attack their pray by injecting toxins to paralyze their victims, and a lot of the targets of these toxins are voltage-gated sodium channels," Yan points out.
Such toxins may act by blocking the channel pore or by preventing shape changes needed to open the channel. To study this, Yan's postdocs tried to visualize the channel bound to different toxins, but couldn't purify enough protein to get good images of the bound toxins. After starting her new lab at Princeton, Yan wanted to pursue the topic in greater depth. In June, her group published a paper in PNAS on the structure of the prototypical bacterial voltage-gated sodium channel, NaChBac.
"We already had a structure for the human channel, so why am I so excited about NaChBac? Because it's a great tool," explains Yan.
Yan's lab used this tool to solve the earlier problem by making a chimeric channel using parts of both NaChBac and Nav1.7. The chimera is easier to purify in large amounts than is the human channel. This made it possible to visualize a tarantula toxin bound to the channel, giving insight into both the toxin's mechanism of action and the channel's.
Now, Yan has a new ambition: to catch a sodium channel in the act of changing shape in response to different membrane voltages. This problem may now be solvable thanks to recent technical improvements, developed by postdoc Yimo Han, that allow for the capture of tiny lipid vesicles called liposomes for cryo-EM.
"Yimo was the first postdoc I recruited at Princeton," says Yan, "Her background is in nanotechnology, so she can teach me a lot."
Encapsulating different ion concentrations inside liposomes should allow scientists to study how ion gradients affect the structures of liposome-embedded ion channels and membrane transporters. Although this work is currently on hold due to the SARS-CoV-2 pandemic, Yan's group is eager to pick up where they left off.
"My postdocs and I have so many ideas to test. We can't wait for the lab to reopen," says Yan.
More information: Shuai Gao et al, Employing NaChBac for cryo-EM analysis of toxin action on voltage-gated Na+channels in nanodisc, Proceedings of the National Academy of Sciences (2020). DOI: 10.1073/pnas.1922903117
Xin Gong et al. Structural Insights into the Niemann-Pick C1 (NPC1)-Mediated Cholesterol Transfer and Ebola Infection, Cell (2016). DOI: 10.1016/j.cell.2016.05.022
Hongwu Qian et al. Structural Basis of Low-pH-Dependent Lysosomal Cholesterol Egress by NPC1 and NPC2, Cell (2020). DOI: 10.1016/j.cell.2020.05.020
Yimo Han et al. High-yield monolayer graphene grids for near-atomic resolution cryoelectron microscopy, Proceedings of the National Academy of Sciences (2019). DOI: 10.1073/pnas.1919114117
Journal information: Cell , Proceedings of the National Academy of Sciences
Provided by Princeton University