October 2, 2019 feature
Quantizing single-molecule surface enhanced Raman Scattering with DNA origami metamolecules
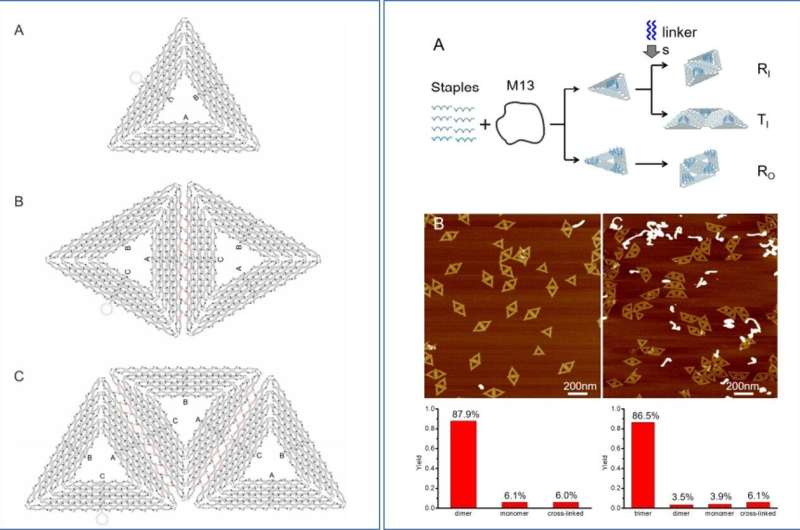
Tailored metal nanoclusters can be actively developed in the lab to manipulate light at the subwavelength scale for nanophotonic applications. However, their precise molecular arrangement in a hotspot with fixed numbers and positions remain challenging to investigate. Weina Fang and colleagues at the school of chemistry and chemical engineering, Key Lab of Interfacial Physics and Technology, Organic Electronics and Information Displays and the Institute of Intelligent Systems in China and Germany; engineered DNA origami metamolecules with Fano resonances (DMFR) (a type of resonance scattering phenomenon), and published the results in Science Advances. The molecules precisely localized single dye molecules to produce quantified surface-enhanced Raman scattering responses (SERS). To deliver tailored plasmonic combinations, Fang et al. developed a general and programmable method by anchoring a set of large gold nanoparticles (L-AuNPs) on prescribed n-tuple (an ordered list of n elements) docking sites of super-origami DNA frameworks.
The research team then built a tetrameric nanocluster with four spatially organized 80-nm L-AuNPs to exhibit peak-and-dip Fano characteristics. They observed the collection of a prominent SERS spectrum at the level of a single dye molecule. The research team expects the DMFR to provide physical insights into single-molecule SERS. The work will open new opportunities to develop plasmonic nanodevices for ultra-sensitive sensing, nanocircuits and nanophotonic lasers.
In nanotechnology, metallic nanostructures that support surface plasmons are of great interest due to their potential to coordinate light at the nanoscale. Metal nanoclusters with spatially coupled nanoparticles known as metamolecules; resemble molecules with spatially coupled atoms to display optical properties attractive for applications as metamaterials. These properties can be included to form nanocircuits, plasmonic sensors and subwavelength waveguides. Theoretical and experimental studies have confirmed that strong field localization at hotspots of plasmonic structures can yield drastic spectroscopic enhancement in the single-molecule regime. Physicists are yet to directly quantify single molecules within hotspots. Challenges include, the simultaneous nanometer-precise control of metal nanoparticle geometries and detecting the number and position of single molecules localized within the hotspot.
Researchers had previously used top-down lithography and bottom-up self-assembly techniques to engineer complex plasmonic nanostructures with high precision to detect single molecules. For instance, DNA origami-based self-assembly can provide a highly programmable approach to design nanopatterns with nanoscale addressability as molecules and nanoparticles. Researchers had already used DNA origami-supported nanoantennas to plasmonically enhance the emission of a fluorophore or Raman dye proximal to metal nanoparticles.
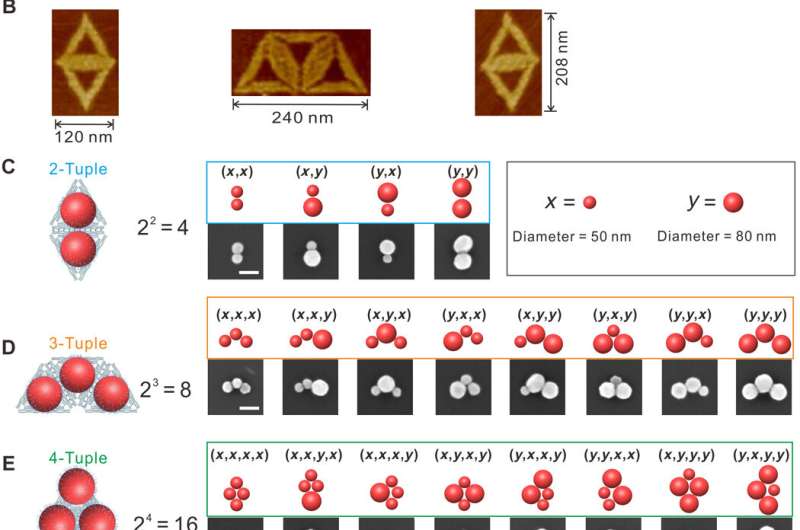
In the present work, Fang et al. reported a general strategy to organize large gold nanoparticles (L-AuNPs) precisely into plasmonic metamolecules with superorigami DNA frameworks. The research team designed the DNA super-origami with n-tuple docking sites to form rhombic tetrameric nanoclusters of AuNPs. They explored the very strong electromagnetic field localized in hotspots at the wavelength of the Fano minimum. Fang et al. developed a platform to quantify surface-enhanced Raman scattering (SERS) of single dye molecules within the hotspot of a DNA origami metamolecule using Fano resonances (DMFR). To tailor plasmonic permutations, the research team used super-origami as templates and anchored L-AuNPs on prescribed n-tuple docking sites.
They constructed three different super-origami templates, with DNA capture strands anchored at specific positions to form rhombus and trapezoid-shaped super-origami structures. The research team anchored a set of L-AuNPs with two different diameters, site-specifically on purified super-origami templates via DNA hybridization. Fang et al. used scanning electron microscopy (SEM) to observe quantitative anchorage of L-AuNPs on the super-origami templates. They noted similarities among several n-tuple structures due to their symmetry and randomness of adsorption to the glass substrate. The scientists observed high-yield formation of tailored L-AuNP plasmon permutations due to several reasons, including:
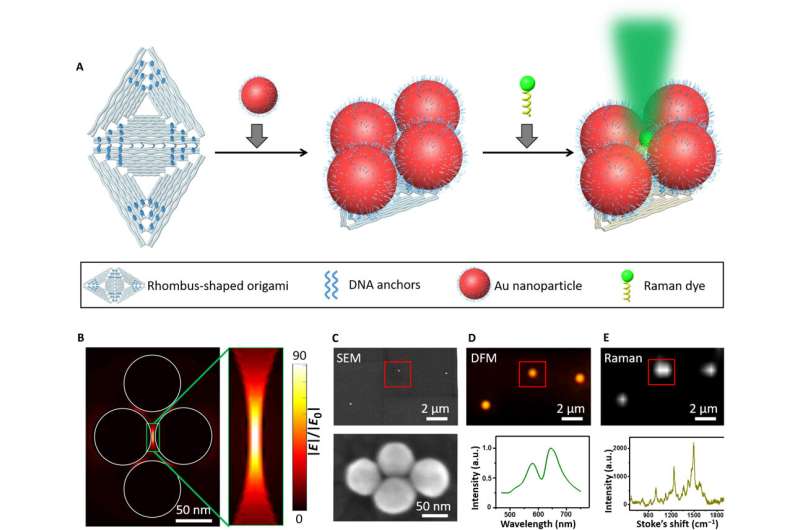
To study structure-correlated optical and plasmonic properties of individual tetramers, Fang et al. used 80-nm AuNP tetrameric clusters. Researchers had previously observed L-AuNPs to exhibit intense absorption and scattering cross-sections. In the present work, the team conducted finite-difference time domain (FDTD) calculations to estimate the size and hotspot region. They observed the electric field in the hotspot region to be 90 times stronger than the field of incident light. Fang et al. immobilized the metamolecules on an indium tin oxide (ITO) glass substrate and confirmed tetrameric morphology of the particles using SEM. The scientists further characterized the scattering and Raman spectra using polarization-dependent dark-field microscopy (DFM) and Raman spectroscopy.
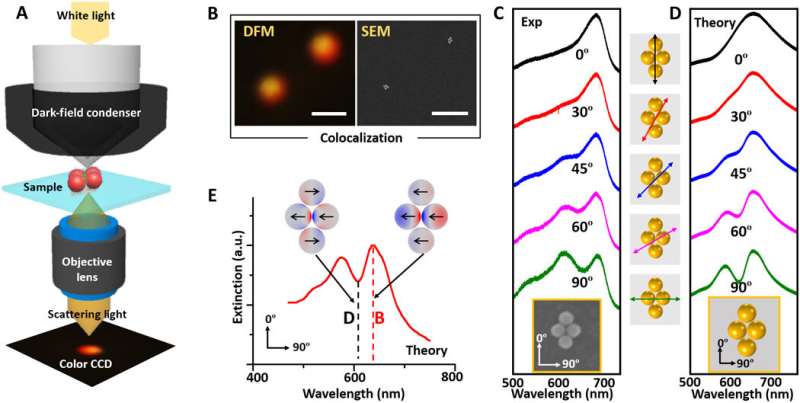
To further investigate the plasmonic properties of individual tetramers, Fang et al. used SEM-DFM correlative imaging. For this, they immobilized the metamolecules on an ITO glass substrate in air and imaged it using an inverted DFM. They observed a narrow and asymmetric dip near 645-nm, as a typical Fano resonance due to the interference between a superradiant "bright" mode and subradiant "dark" mode in the setup. The research team observed a similar trend in incident light orientation-dependent spectra evolution using finite element simulation software (COMSOL). The experimental and calculated Fano minimums differed slightly, due to DNA coating and the origami substrates.
Having experimentally confirmed the DMFR (Fano resonances) from tetrameric metamolecules, Fang et al. explored their potential for SERS analysis, using a DNA binding dye SYBR Green I to study structure-correlated Raman properties. After intercalating the green dye on DNA bound on L-AuNPs and the DNA origami template, they used SEM-Raman colocalization to measure Raman enhancement from tetrameric metamolecules. To better understand the phenomenon, they compared a symmetric tetramer with a distorted asymmetric tetramer. The integrity of the symmetric electric field was broken in the distorted metamolecule. In comparison, Fano-like resonances observed in the well-formed tetramer resulted in high SERS electric enhancement.
The scientists also quantitatively studied the metamolecules at the single-molecule level using a ROX (carboxy-X-rhodamine) molecule as the Raman dye. They deliberately anchored ROX molecules in the hotspot region of the tetrameric cluster and observed the SERS intensity to increase quantitatively with the number of ROX molecules and saturate upon accommodation of up to six ROX molecules. Importantly, the team could specifically detect the Raman signal at the scale of a single ROX dye molecule.
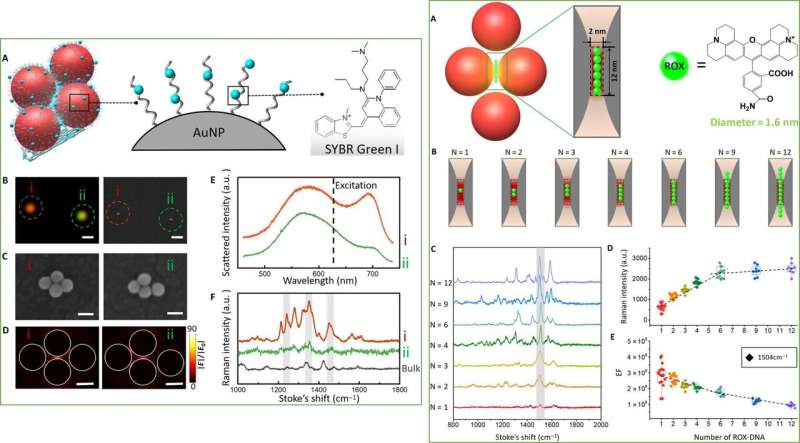
In this way, Weina Fang and co-workers demonstrated the use of super-origami DNA frameworks as a general method to fabricate plasmonic nanostructures. They successfully constructed metamolecules with DMFR to quantitatively analyze Raman enhancement localized in the hotspot. The results provided direct evidence on single-molecule SERS. The research team engineered super-origami metamolecules with strong plasmonic enhancement as an ideal platform to study single molecule biophysical studies and ultrasensitive sensing. The team envision applications of the flexible origami construction for a variety of targets in nanoelectronics, nanophotonics and biosensing.
More information: Weina Fang et al. Quantizing single-molecule surface-enhanced Raman scattering with DNA origami metamolecules, Science Advances (2019). DOI: 10.1126/sciadv.aau4506
N. Engheta. Circuits with Light at Nanoscales: Optical Nanocircuits Inspired by Metamaterials, Science (2007). DOI: 10.1126/science.1133268
Eva-Maria Roller et al. Hotspot-mediated non-dissipative and ultrafast plasmon passage, Nature Physics (2017). DOI: 10.1038/nphys4120
Journal information: Science Advances , Science , Nature Physics
© 2019 Science X Network