Researchers use an optical technique to probe magnetism at a hidden interface between two exotic thin films
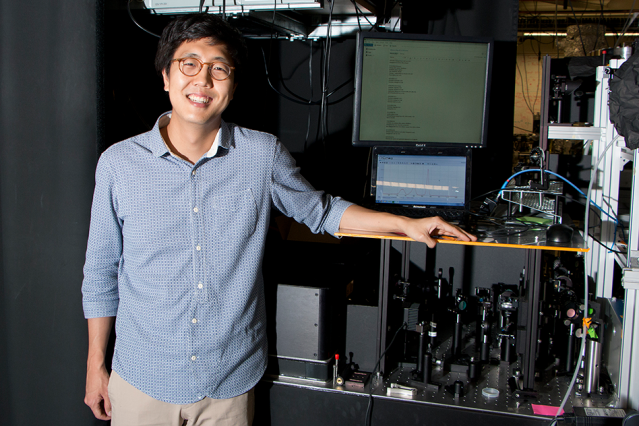
Magnetic properties of materials underlie technologies from old-fashioned recording tape to modern hard drives, and scientists are constantly pushing to develop new uses from magnetic behavior. Recently, researchers at MIT figured out how to use light pulses to map the magnetic direction and strength of a buried interface between two exotic materials, bismuth selenide and europium sulfide.
In a sandwich of extremely thin layers of these materials, one distinguished by electrical conduction only on it surface, the other by becoming magnetic at extremely low temperatures, Nuh Gedik, the Lawrence C. (1944) and Sarah W. Biedenharn Career Development Associate Professor of Physics, and graduate student Changmin Lee measured the magnetism at the interface of the two materials.
Their technique relies on a transformation that occurs at low temperature under an applied magnetic field as intense red laser light pulses pass through the sample and a small fraction of red light photons fuse together to form a single blue light photon with twice the energy of a single red photon. This transformation, known as magnetic second harmonic generation, occurs only at low temperatures.
Even though these materials are extremely thin, ranging from 1 to 10 nanometers, the second harmonic generation technique is able to selectively probe magnetism just at the interface of the bismuth selenide and europium sulfide sample, while ignoring any interference from the bulk of the material. "That's really the beauty," Gedik says.
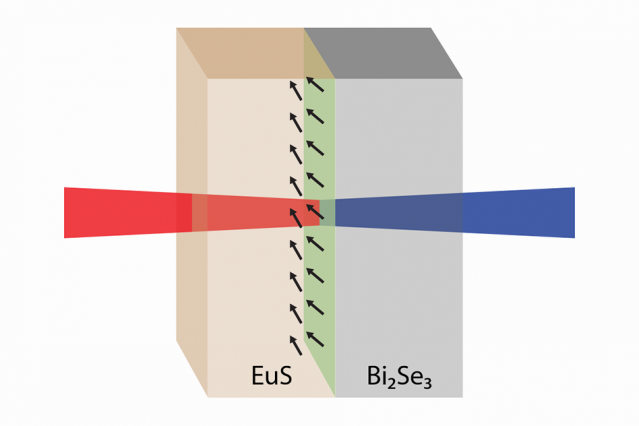
Their results were published in Nature Communications. Lee performed the optical measurements and analyzed the data while Gedik supervised the optical measurements. Co-authors include MIT postdoc Ferhat Katmis, who prepared the thin film samples, and Mitsui Career Development Associate Professor of Physics Pablo Jarillo-Herrero, and Department of Physics senior research scientist Jagadeesh S. Moodera, who supervised the thin film growth.
Their experimental setup probed the sample with ultrafast femtosecond laser pulses, varying the orientation of a magnetic field either parallel or perpendicular to the sample, and collected data on blue light emission from the sample. They collected data as they rotated the sample through a full circle relative to the light source. (They also filtered out unaltered red light.)
In Figure 2 in the slideshow above, the six-fold lobes, similar to flower petals, show how the intensity of blue light coming out of a thin film of europium sulfide/bismuth selenide changes as the sample is rotated. Measuring this blue light, or second harmonic generation, reveals the direction and strength of magnetism buried at the interface between europium sulfide and bismuth selenide.
Besides being sensitive to magnetism at the interface between the materials, this magnetic second harmonic generation approach enables measuring both parallel and perpendicular magnetic directions at the same time and holds promise for spatial imaging of magnetic domains in the future, Gedik says.
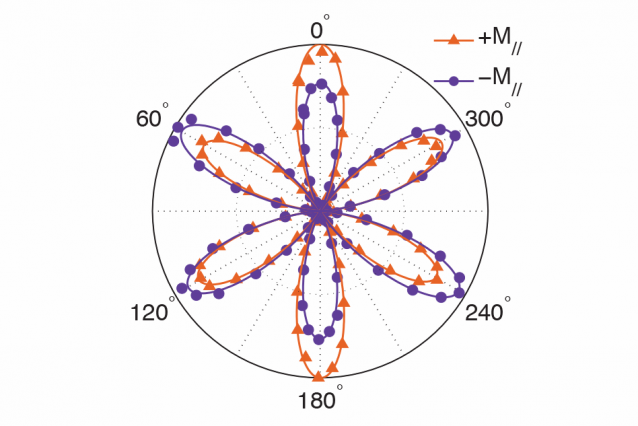
The study paired europium sulfide, a ferromagnetic insulator (FMI), with bismuth selenide, a topological insulator (TI). While the researchers were able to measure the transformation of light caused by interaction with magnetism in their sample, the mechanism is not yet fully understood.
In a ferromagnetic material such as europium sulfide, its magnetic domains normally are randomly distributed, with some magnetic regions pointing up, or North, and some pointing down, or South. Applying a strong external magnetic field orients all of the magnetic regions in the same direction, which is how the researchers conducted these studies.
"Here we are just focusing on one part of the sample and measuring the collective magnetization at that point, but eventually we would like to put a [charge-coupled device] after the sample so that we can actually visualize spatially what the magnetic moments are within that spot. When we actually do that, without applying any magnetic field, we will be able to distinguish where all these magnetic moments are aligned," Lee explains.
The researchers are interested to learn more about a theoretically predicted state called the "chiral edge state," which is a one-dimensional current that flows at the two-dimensional interface between europium sulfide and bismuth selenide. In this state electrons align themselves according to a property called spin. "Our goal is to extend this technique so we can actually visualize what the spin texture looks like at the interface between a ferromagnet and topological insulator, and I think that's what's really unique about this technique," Lee says.
If they are successful in pairing this technique with a digital recording device, they may be able to detect this exotic conducting state that is expected to exist at the boundary between these two materials, Gedik suggests. Such a dissipation-less (zero resistance) one-directional current could be useful for future spintronic device applications.
More information: Changmin Lee et al. Direct measurement of proximity-induced magnetism at the interface between a topological insulator and a ferromagnet, Nature Communications (2016). DOI: 10.1038/ncomms12014
Journal information: Nature Communications
Provided by Massachusetts Institute of Technology