Clarifying the convoluted chemical dance that charges fuel cells
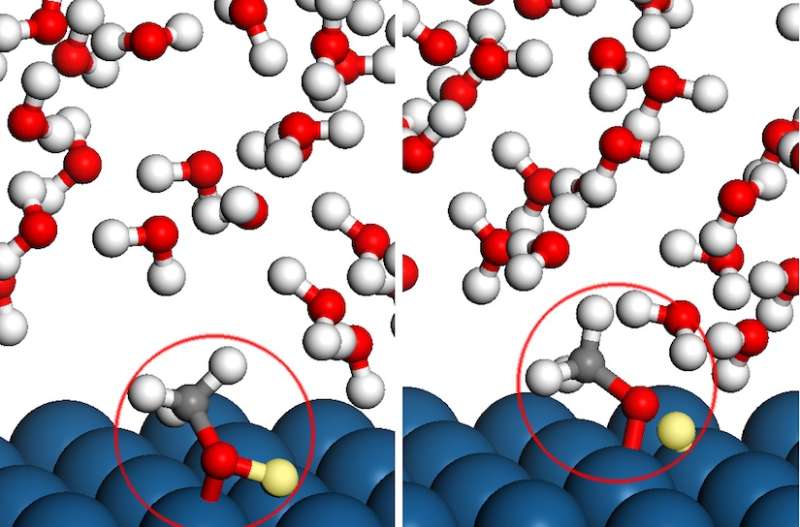
Using a unique combination of advanced computational methods, University of Wisconsin-Madison chemical engineers have demystified some of the complex catalytic chemistry in fuel cells—an advance that brings cost effective fuel cells closer to reality.
"Understanding reaction mechanisms is the first step toward eventually replacing expensive platinum in fuel cells with a cheaper material," says Manos Mavrikakis, the Vilas Distinguished Achievement Professor and Paul A. Elfers Professor in chemical and biological engineering at UW-Madison.
Mavrikakis and colleagues at Osaka University, Japan, published details of the advance in the journal Proceedings of the National Academy of Sciences August 8, 2016.
Fuel cells generate electricity by combining electrons and protons with oxygen from the air to form water. Those electrons and protons come from a chemical fuel such as methanol. To make the reaction that generates protons faster, fuel cells typically contain catalysts. With the right catalyst and enough fuel and air, fuel cells could provide power very efficiently.
Someday, fuel cells could make laptop batteries obsolete. Mere tablespoons of methanol could potentially provide up to 20 hours of continuous power. But alternatives to the expensive platinum catalyst in today's fuel cells haven't emerged because scientists still don't fully understand the complicated chemistry required to produce protons and electrons from fuels.
And finding a good catalyst is no trivial task.
"People arrived at using platinum for a catalyst largely by trial and error, without understanding how the reaction takes place," says Mavrikakis. "Our efforts developed a big picture of how the reaction is happening, and we hope to do the same analysis with other materials to help find a cheaper alternative."
At first glance, the chemistry sounds straightforward: Methanol molecules awash in a watery milieu sit down on a platinum surface, then give up one of their four hydrogen atoms. Electron from that hydrogen atom spark electric current.
In reality, the situation is not so simple.
"All of these molecules, the water and the methanol, are actually dancing around the surface of the catalyst and fluctuating continuously," says Mavrikakis. "Following the dynamics of these fluctuating motions all the time, and in the presence of an externally applied electric potential, is really very complicated."
The water molecules are not mere wallflowers sitting on the sidelines of the methanol molecules reacting with platinum; rather, they occasionally cut in to the chemical dance. And varying voltage on the electrified surface of the platinum catalyst tangles the reaction's tempo even further.
Previously, chemists only simulated simplified scenarios—for example, fuel cells without any water in the mix, or catalytic surfaces that didn't crackle with electricity. Unsurprisingly, conclusions based on such oversimplifications failed to fully capture the enormous complexity of real-world reactions.
Mavrikakis and colleagues combined their expertise in two powerful computational techniques to create a more accurate description of a very complex real environment. They first used density functional theory to solve for quantum mechanical forces and energies between individual atoms, then built a scheme upon those results using molecular dynamics methods to simulate large ensembles of water and methanol molecules interacting among themselves and with the platinum surface.
The detailed simulations revealed that the presence of water in a fuel cell plays a huge role in dictating which hydrogen atom breaks free from methanol first—a result that simpler methods could never have captured. Electric charge also determined the order in which methanol breaks down—surprisingly switching the preferred first step at the positive electrode.
This type of information enables scientists to predict which byproducts might accumulate in a reaction mixture, and select better ingredients for future fuel cells.
The results represent the culmination of six years of effort across two continents. Jeffrey Herron, the first author on the paper, started developing the methodologies during a summer visit to work under the paper's second author, Professor Yoshitada Morikawa in the Department of Precision Science and Applied Physics at Osaka University, Japan.
Herron, who completed his PhD in 2015 and is currently a senior engineer for The Dow Chemical Company, further refined these approaches under Mavrikakis' guidance over several subsequent years in Madison.
"A lot of work over many years went into this paper," says Mavrikakis. "The world needs fuel cells, but without uwww.pnas.org/content/early/recentnderstanding how the reaction takes place, there is no rational way to improve."
More information: Jeffrey A. Herron et al. Ab initio molecular dynamics of solvation effects on reactivity at electrified interfaces, Proceedings of the National Academy of Sciences (2016). DOI: 10.1073/pnas.1604590113
Journal information: Proceedings of the National Academy of Sciences
Provided by University of Wisconsin-Madison