Interaction of tailored light with a single atom and individual nanostructures
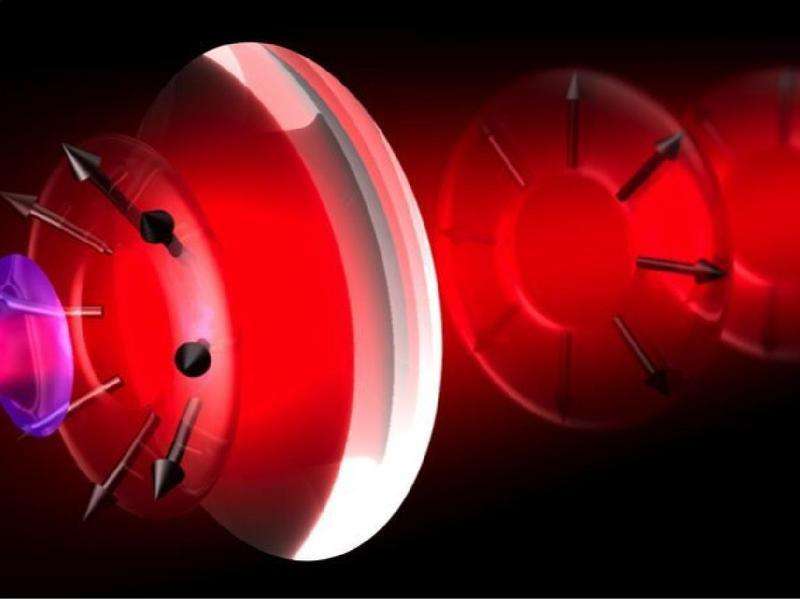
By adapting a mode of the light field to a system under study, the interaction of light with matter can be optimized. In this context, the spatial distribution of the electric field of such a tailored mode plays an important role. At the MPI for the Science of Light, this approach is utilized to couple light to a single atom or individual nano-particles. It was shown, for example, that light can be coupled to an ion trapped in a parabolic mirror with high efficiency. In other studies, the scattering behavior of individual nano-particles was controlled using polarization tailored light.
Individual atoms, ions and molecules form the fundamental building blocks of our world and the universe as a whole. We perceive the materials formed from these building blocks through their interaction with light, among other things. More complex objects, such as nanostructures, can be characterized and also manipulated by means of their specific interaction with the light field. This manipulation forms the basis for a many potential applications, e.g. in biophysics or quantum information processing. Furthermore, individual nanostructures can themselves be the building blocks of so-called metamaterials.
The efficiency of the interaction between light and individual atoms or nanostructures scales with the adaption of the light field to the object under study. This tailoring extends far beyond selecting the correct wavelength for the light. The precise shaping of the temporal and spatial distribution of the intensity of the light and the polarization vector, i.e. the direction of oscillation of the electric field, is thus crucial in all examples discussed here. This mode matching leads to a light field being concentrated to dimensions that are smaller than the wavelength of the light when focussed onto the target object.
The generation of longitudinal electric fields, which couple efficiently to a suitably oriented linear electric dipole that can be realized by a single atom or a suitable nanostructure can be given as an example of the use of tailored light fields. It requires a calculation done of how exactly the field mode emitted by such a dipole is transformed by the optical focussing system used in the experiment. For the example presented here, this procedure results in a mode with annular intensity distribution and radially aligned polarization vector (Fig. 1).
Generation of a dipole mode to couple to an atom
If a radially polarized ring mode is focused with a parabolic mirror that is far deeper than its focal length, it is possible to generate an almost perfect dipole wave, which propagates towards the focus of the mirror from nearly the whole solid angle. This configuration maximizes the efficiency of the interaction between the light field and an atom in free space provided the atom is localized at the focal point of the mirror . In the experiment, it was possible to generate a tailored, radially polarized mode, which matches the ideal field distribution to 98% (cf. Fig. 2(a)).
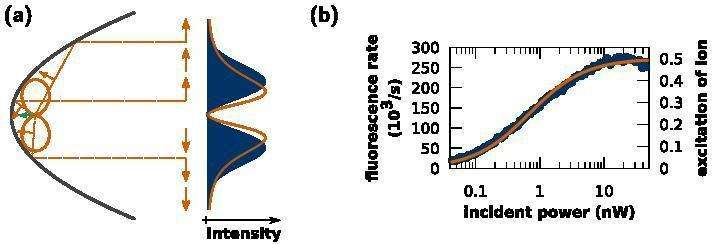
A single ion in a parabolic mirror
An essential part of the task of coupling a light mode to a single atom with maximum efficiency is to place the atom at the intensity maximum of the focussed mode in a controlled way. This is realized in the present case by using a singly charged ytterbium ion as the atom. Ions can be accurately positioned and trapped over long periods by using a suitable electrode arrangement to which an AC voltage is applied. For this purpose an ion trap was developed which is specially adapted to the parabolic mirror geometry and shadows the ion from the focussed light as little as possible.
A deep parabolic mirror as described above is not only particularly suitable for focussing light onto an atom. As symmetry arguments suggest, the light emitted from the atom can also be efficiently collected by such a mirror. This has been demonstrated for the ytterbium ion with a 54% yield of the emitted light particles. This setting can be thought of as a spotlight whose filament is a single ion.
Efficient coupling of individual ions to the light field
So-called saturation measurements are particularly suitable for characterizing the coupling of the ion to the electromagnetic field. These are measurements where the degree of excitation of the atom is determined by varying the power of the light focussed onto the atom. The quality of the coupling can be determined directly by means of a comparison with the power which would be required for 100% coupling efficiency under ideal conditions. The efficiencies achieved with the parabolic mirror arrangement are among the highest that have been demonstrated to date for individual quantum objects in free space.
Tailored light as a tool to investigate individual nanostructures
As has already been mentioned above, the spatial distribution of the intensity, polarization and phase of the electromagnetic field is extremely important in the interaction of sub-wavelength structures with light. This fact can be cleverly exploited by tailoring the spatial distribution of the parameters of the light, which is to be used to investigate the optical properties of individual nanostructures. Focussing this light creates for example a three-dimensional electric field distribution of the order of the wavelength (Fig. 1). The sub-wavelength nanostructure to be investigated (Fig. 3 (a)) thus has a large overlap with the incident light field, but is still significantly smaller than the focal spot (Fig. 1). The interaction between the nanostructure and the focal field distribution is now strongly dependent on where the nanostructure is positioned relative to the optical axis in the focal plane. Resonances can thus be selectively excited and many coupling scenarios can be realized, and switching between them is achieved by a simple displacement of the sample in the focal plane. When the focal field distribution is accurately known, the optical properties of the nanostructure can be investigated and accurately determined by measuring the scattered light.
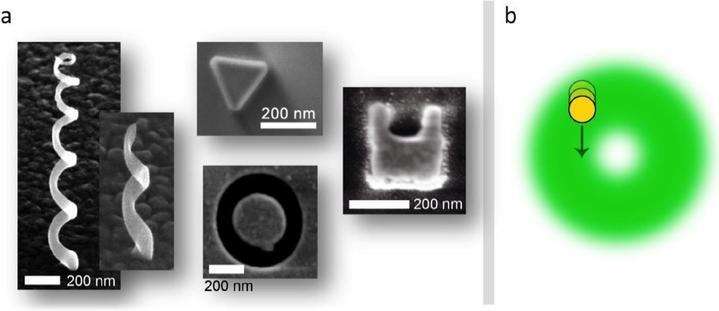
This methodology has already been very successfully applied to the investigation of numerous nanostructures and nanoscopic objects (Fig. 3(a)). Nanostructures which have been investigated and characterized in this way can themselves act as building blocks of new types of materials, so-called metamaterials, with extraordinary properties, when arranged in two- or three-dimensional fields where the separation of adjacent neighbours is also smaller than the light wavelength. The material appears to be homogeneous, just like naturally occurring materials. With the aid of these extraordinary, artificial materials, light can be guided around objects and reflections can be suppressed, as happens with an invisibility cloak, the transmission through ultrathin layers can be greatly reduced or further interesting applications realized.
Experimental field measurement of strongly focussed light
A further application of the interaction between tailored light and individual nanostructures is also the high-accuracy measurement of the distribution of the light itself and its properties with spatial resolutions far below the optical wavelength. This requires a nanostructure that has been characterized in detail, and which, in this scheme, acts as a probe that is scanned through the field distribution under investigation line by line (Fig. 3(b)). Combining this with a sophisticated measurement and evaluation methodology allows for measuring even very small focal spots. This enables the reconstruction of complete three-dimensional electric field distribution, including the strength (amplitude) or the relative phase relationship of the individual field components. Phase information is obtained by exploiting the interference of the scattered light with a reference beam, represented here by the incident light itself, similar to standard interferometry. Minute spatial variations of the field can be made visible in this way. The methodology can be used to investigate the quality of optical focussing systems such as microscope objectives and their aberration.
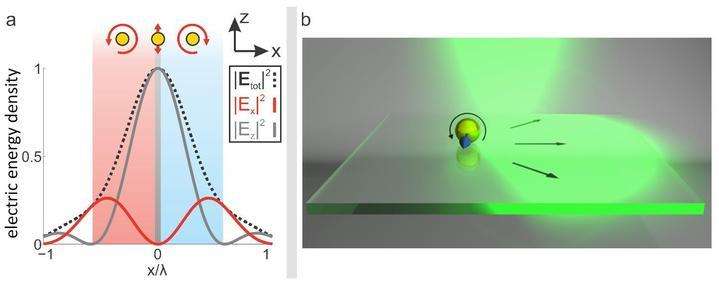
Polarization-controlled, directive scattering and waveguide coupling
The controlled manipulation of the spatial degrees of freedom of the light also opens up the possibility of steering the scattering off a nanoparticle. Depending on the local field with which the particle interacts, it is thus possible to control the direction and degree of directedness of the scattering in a suitable system. To this end, the scattering particle must simply be placed on a substrate or waveguide whose surface breaks the symmetry of the system in the forward direction. A spinning dipole can be induced in the particle for a suitable distribution of the excitation field, e.g. for strongly focussed, radially polarized light beyond the optical axis (Fig. 4(a)). The spinning axis of the induced dipole is aligned parallel to the substrate surface, while its orientation depends on the position of the particle in the beam. Such a nanobeacon enables directional and controllable light coupling into optical waveguides or systems of optical waveguides (Fig. 4(b)). For instance, this paves the way for high-precision control of light propagation in optical networks.
More information: "Efficient saturation of an ion in free space." Applied Physics B December 2014, Volume 117, Issue 3, pp 797-801 DOI: 10.1007/s00340-014-5817-y
"Nanointerferometric amplitude and phase reconstruction of tightly focused vector beams." Nature Photonics 8, 23–27 (2014) DOI: 10.1038/nphoton.2013.289
"Polarization Tailored Light Driven Directional Optical Nanobeacon." Nano Lett., 2014, 14 (5), pp 2546–2551 DOI: 10.1021/nl5003526
Journal information: Nature Photonics , Nano Letters
Provided by Max Planck Society