April 20, 2021 feature
Holographic metasurface gas sensors for instantaneous visual alarms
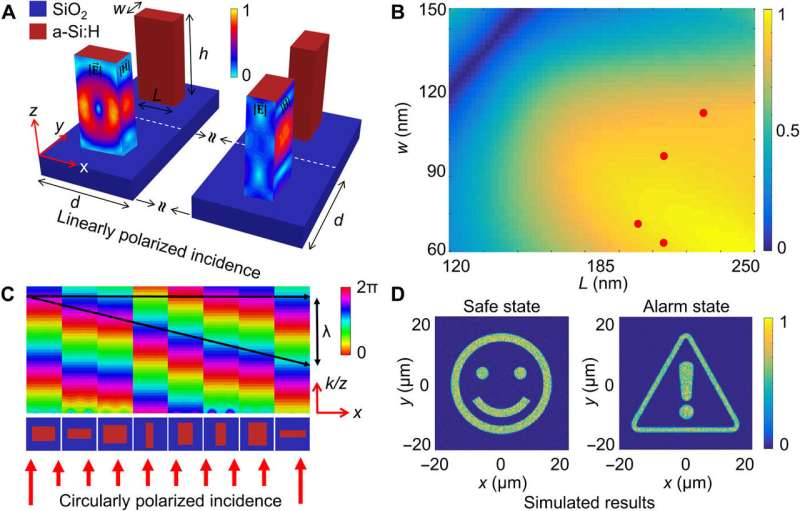
Biological and chemical substances can be rapidly detected in real-time for public health and environmental monitoring purposes. In a new report now on Science Advances, Inki Kim and a research team in mechanical engineering, materials science and electrical engineering in the Republic of Korea and in Pakistan proposed a compact sensor platform to integrate liquid crystals (LCs) and holographic metasurfaces to sense the existence of a volatile gas, and then provide an immediate visual holographic alarm. The team combined the setup to form ultracompact gas sensors without complex instruments in order to detect gas via visual cues. The researchers proved the applicability of the compact sensors by integrating the metasurface-based gas sensor on safety goggles via a one-step nanocasting process.
Liquid crystal integrated metasurfaces
Materials scientists have devised a variety of methods to detect target substances and their corresponding sensor platforms including electrical, optical and radio-frequency or microwave signal changes. Among the sensors, liquid crystal-based sensors are suited due to their sensitivity and rapid detection in real-time. In this work, Kim et al. proposed a compact sensor platform that combined liquid crystals with holographic metasurfaces known as LC-integrated metasurfaces (LC-MS) to sense a volatile gas and provide instant feedback via a visual holographic alarm. The method integrated the advantages of the stimuli responsiveness of liquid crystals and the compactness of metasurfaces, while maximizing the effectiveness of the sensor by providing gas sensing conformation. The team developed the metasurface hologram using hydrogenated amorphous silicon (a-Si:H) designed to reproduce different holographic images based on the geometric and propagation phase of each nanostructure. Depending on the presence or absence of volatile gases, the setup could transmit different polarization states of light.
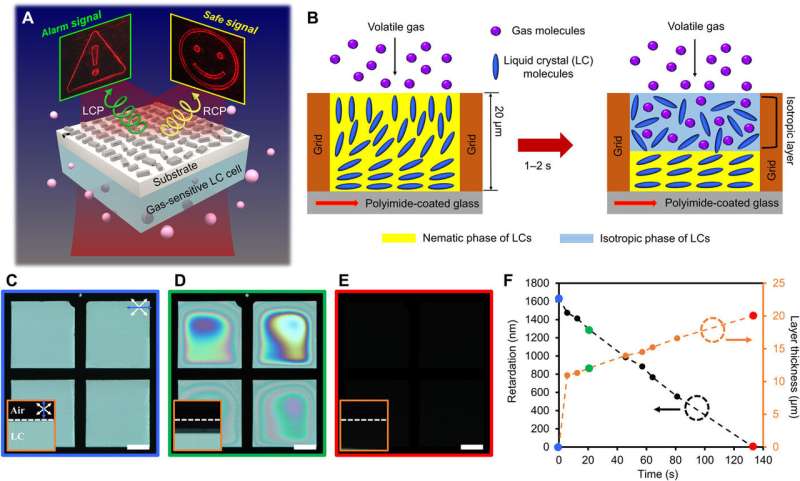
Designing gas-responsive liquid crystal cells
The team regulated the molecular order of liquid crystals through a variety of external stimuli. Kim et al. first observed and characterized the gas responsiveness of LCs in the simplest geometry. To accomplish this, they filled a microwell structure with nematics (relating to, or being the phase of a liquid crystal). During the experiments, the scientists used an isopropyl alcohol (IPA) gas as a target hazardous gas for detection. When they exposed IPA gas at a constant concentration in a closed chamber cell, it transitioned from white to colored. The results indicated the capability of the LC cell to promptly detect toxic gases. The team then conducted experiments with a range of gases with diverse dose conditions to measure detection time-frames at around 1.3 seconds for chloroform, 1.6 seconds for acetone, 13.9 seconds for IPA gas and 58.3 seconds for methanol. With higher doses, they observed faster response rates.
Designing spin-encoded meta-holograms with asymmetric spin-orbit interaction.
The scientists designed the spin-encoded metasurface based on the conventional Pancharatnam-Berry (PB)-phase modulation method to understand the symmetry inherent to spin and degrees of interaction. The resultant total efficiency of the device was only 50 percent. To overcome optical energy loss, the team designed the metasurface via spin-encoding through asymmetric coupling to function for the left circularly polarized (LCP) light and right circularly polarized (RCP) light to help break the conventional efficiency limit. The confinement of magneto-electric resonances within the nano-antennas validated the optimization procedure. The team selected the size of the nanoantennas depending on their ability to retain a high transmission efficiency and a fixed incremental phase shift. They developed holograms for safe (smiley face) and alarm states (exclamation mark) obtained from the designed asymmetric coupled metasurface. To validate the functionality of the asymmetric coupled metasurface, Kim et al. numerically simulated a metahologram with commercially available full-wave electromagnetic simulation software—Lumerical Inc.
Holographic gas sensors and wearable applications
The scientists visualized real-time gas exposure using the gas responsive LC-MS system. They then tested the sensing capacity, the fast switching rates of the holographic image and high diffraction efficiency of the gas sensor in the optical setup on exposure to a volatile gas. Kim et al. used a ubiquitous volatile gas source i.e., a board marker pen containing various organic solvents including IPA (isopropyl alcohol). The metahologram devices contained an a-Si:H nanoantenna. In the absence of the volatile gas, the sensor projected a smiling holographic image as a safety sign. Upon gas exposure, the sign instantly switched to an exclamation mark to provide an 'alarm sign." This process occurred when volatile gases from the pen diffused into the liquid crystal layer, which reduced the optical retardance to convert polarization of the output polarization beam from RCP (right circularly polarized light) to LCP (left circularly polarized light). When the team removed the gas, the hologram rapidly restored itself to its safety sign, as the liquid crystals returned to their initial orientation. The process could occur within a few seconds and the distance of the marker from the sensor did not affect the response time. This type of sensor will have applications to detect the exposure of harmful gases during transportation or gas-sensitive product storage. The team can also extend the application by developing wearable devices based on flexible metasurfaces formed via a one-step nanocasting process. Unlike conventional nanoprinting, Kim et al. included a functionalized ultraviolet (UV)-curable resin with titanium oxide nanoparticles as a resin composite for use as a dielectric metasurface during the process, without using complex processes of nanofabrication. This process is also suited for mass-production manufacture.
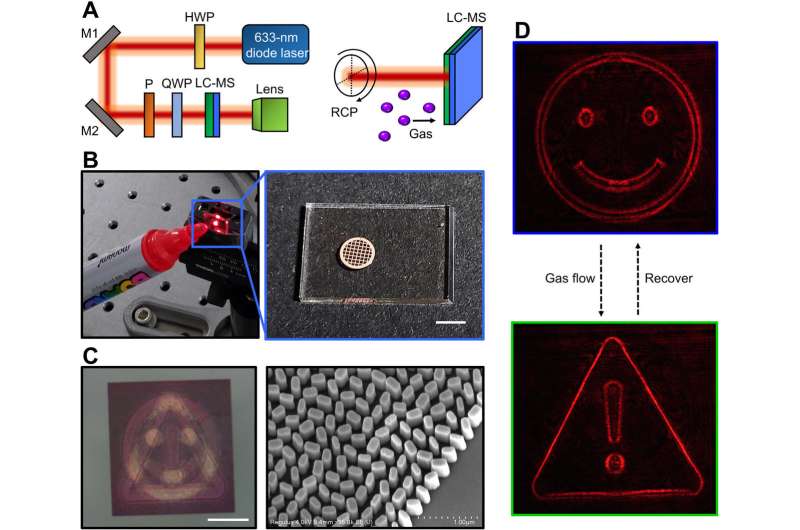
Proof-of-concept
As proof of concept, they then printed a flexible and conformal holographic gas sensor on a flexible polyethylene terephthalate (PET) film and attached that to the surface of safety goggles. The team then optimized parameters of the nanoparticle-resin composite metasurface for 532 nm wavelength incident light, although the construct also functioned across a broader range of wavelengths. In this way, Kim et al. developed a clear holographic alarm. In the future, they can miniaturize and integrate the proposed flexible and conformal gas sensor to fully establish wearable and compact gas sensors. These sensors functioned without any additional complex mechanical and electronic devices to enable low-cost wearable gas sensors that can be integrated in factories, construction and cleaning applications. The device can also function in reflective mode by using ambient light instead of an internal light source to develop cheaper, simpler and miniaturized sensor platforms.
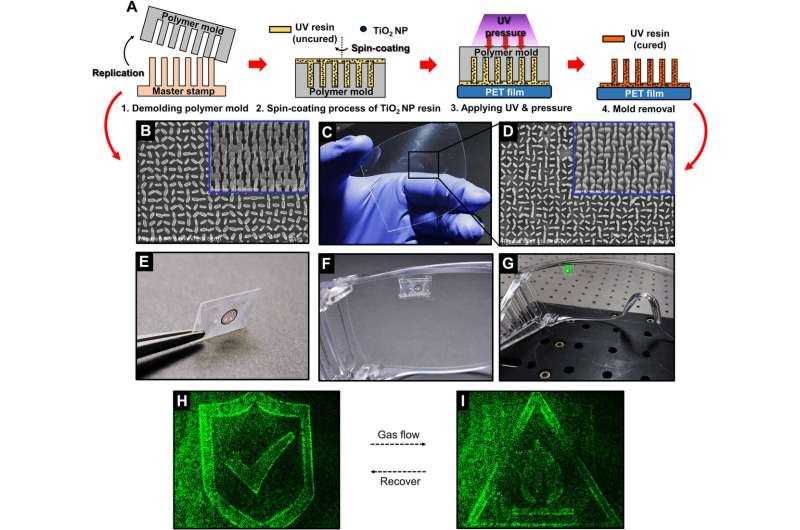
Outlook
In this way, Inki Kim and colleagues proposed general and versatile design rules to realize the potential of dynamically tunable and stimuli-responsive metasurface systems. The proposed LC-MS gas sensor platform provided a rapid visual alarm system suited to detect toxic gases, the team verified the designed gas sensors relative to their practicality and feasibility to form an ultracompact, cost-effective and user-friendly gas sensor system that worked without complex requirements. The system is applicable as wearable sensors to prevent gas poisoning accidents where the sensor can be mounted onto gloves or glasses to give a prompt visual warning via holographic alarms.
More information: Kim I. et al. Holographic metasurface gas sensors for instantaneous visual alarms, Science Advances, DOI: 10.1126/sciadv.abe9943
Wu C. et al. Fano-resonant asymmetric metamaterials for ultrasensitive spectroscopy and identification of molecular monolayers. Nature Materials doi.org/10.1038/nmat3161
Kinkhabwala A. et al. Large single-molecule fluorescence enhancements produced by a bowtie nanoantenna. Nature Photonics, doi.org/10.1038/nphoton.2009.187
Journal information: Science Advances , Nature Photonics , Nature Materials
Provided by Science X Network
© 2021 Science X Network