Quantum-entangled light from a vibrating membrane
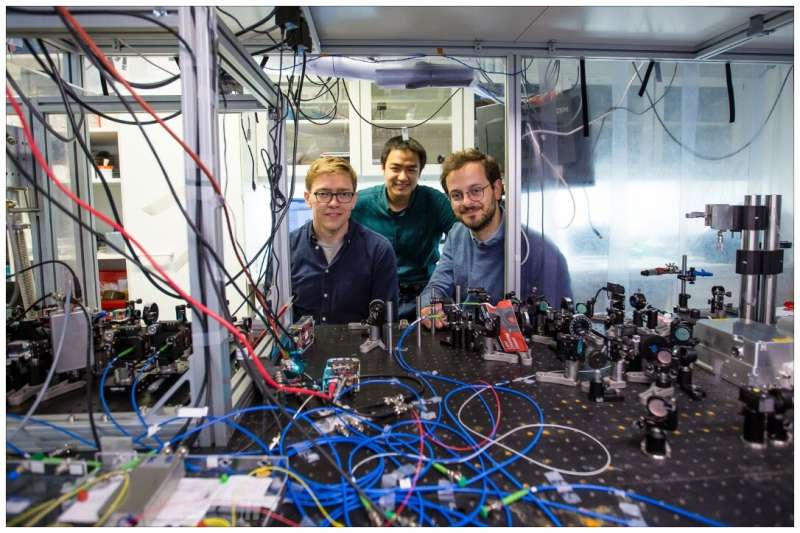
Entanglement, a powerful form of correlation among quantum systems, is an important resource for quantum computing. Researchers from the Quantum Optomechanics group at the Niels Bohr Institute, University of Copenhagen, recently entangled two laser beams through bouncing them off the same mechanical resonator, a tensioned membrane. This provides a novel way of entangling disparate electromagnetic fields, from microwave radiation to optical beams. In particular, creating entanglement between optical and microwave fields would be a key step towards solving the long-standing challenge of sharing entanglement between two distant quantum computers operating in the microwave regime. The result is now published in Nature Communications.
In a future quantum internet, that is the internet of quantum computers, entanglement needs to be shared between two distant quantum computers. This is typically done with electromagnetic links like optical fibers. Presently, one of the most advanced quantum systems is based on superconducting circuits, which work in the microwave regime. As advanced as it is, connecting such computers in networks still poses a steep challenge: microwaves can't propagate far without loss which is harmful to quantum computing tasks. One way of alleviating this problem is to first entangle microwaves with optical fields, then use optical links, with far lower loss, for long-distance communication. However, due to large difference in wavelengths (millimeters for microwaves and micrometers for light), this conversion remains a challenge.
Objects vibrate when bombarded by light particles
When an electromagnetic field, i.e. a laser beam, is reflected off a vibrating object, it can read out the vibration. This is a widely used effect in optical-based sensing. On the other hand, an electromagnetic field is composed of photons, energy bullets of light. As the light is bounced off the object, the photons bombard it, leading to additional vibration. This additional vibration is called quantum backaction. Reflection of two electromagnetic fields upon the same mechanical object provides an effective interaction between the fields. Such interaction takes place irrespective of the wavelength of the two fields. Then, this interaction can be exploited to create entanglement between the two fields, independently of their wavelengths, e.g. between microwave and optics. Though quantum backaction can be prominent for objects as small as an atom, only in recent years, researchers have been able to make macroscopic mechanical devices that are so sensitive to observe this effect.
Ultra-sensitive mechanical device mediates entanglement
In their now reported work, researchers from the Quantum Optomechanics group use a thin membrane, 3x3 mm wide, made of silicon nitride and pierced with a pattern of holes that isolates the motion of the central pad. This makes the device sensitive enough to show quantum backaction. They shine two lasers on the membrane simultaneously, where one laser sees the quantum backaction of the other, and vice versa. In this way, strong correlations, and indeed entanglement, is generated between two lasers. "You could say that the two lasers 'talk' through the motion of the membrane," says Junxin Chen, who has been working on the project during his Ph.D., and is one of the primary authors of the scientific article.
"The membrane oscillator functions as an interaction media, because the lasers don't talk to each other directly—the photons don't interact themselves, only through the oscillator." Junxin Chen further says, "the interaction between photons and the membrane is wavelength independent, allowing in principle microwave-optical entanglement." Further experimental work will be necessary to do this—in particular operation of the membrane at a temperature close to absolute zero, at which superconducting quantum computers work today. Experiments along these lines are underway at the Niels Bohr Institute.
More information: Junxin Chen et al, Entanglement of propagating optical modes via a mechanical interface, Nature Communications (2020). DOI: 10.1038/s41467-020-14768-1
Journal information: Nature Communications
Provided by University of Copenhagen