Scientists show how water molecules add unwanted stability
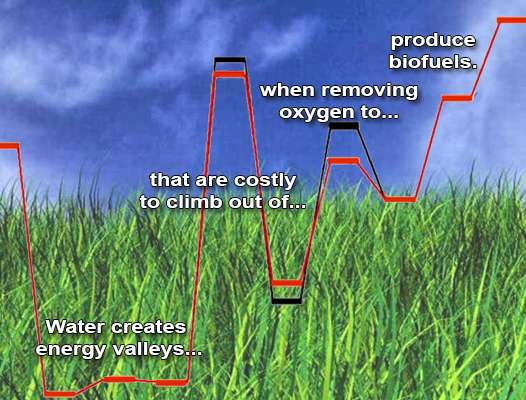
In creating fuels from agricultural waste and other biomass, water slows a solid acid catalyst's ability to get the job done, costing time and energy. But scientists didn't know how the adverse reactions occurred. A team from Pacific Northwest National Laboratory and a major German university delved into the mystery.
Because removing oxygen is crucial for biofuel production, the team focused on how water interferes with two oxygen-removal paths driven by a zeolite catalyst. Water blocks both pathways. The team demonstrated unequivocally that water helps create a highly stable intermediate. Turning this intermediate into the desired product is like pushing a boulder uphill. It takes far more energy than if the water hadn't interfered. And when it comes to producing fuels, adding energy is expensive in economic and environmental terms.
"Water is ubiquitous and abundant in biomass-derived feedstocks," said Dr. Hui Shi, a postdoctorate fellow who has worked for 3 years at PNNL and participated in this joint research. "Understanding water's impact is crucial to the design and synthesis of catalytic materials that are less or not negatively affected by water."
To upgrade renewable biomass sources into liquid fuels requires removing oxygen atoms, often from intermediately formed alcohols. When catalyzed by acids, this process is known as dehydrating an alcohol. The team's detailed analysis of two model dehydration pathways could aid others in engineering subtle adjustments to reduce biofuel production's costs.
The team's work shows how to gather the needed information. "Researchers really need to combine experiments and theory to build models that fully describe the entire reaction, however simple it may seem," said Dr. Donald Camaioni, who is mentoring Shi as part of the postdoctoral program. "It pays off when it comes to rational catalyst and process design, which ought to be more knowledge based than empirical."
To achieve a molecular-level understanding of the two pathways, known as the "monomer path" and the "dimer path," they used rigorous kinetic, calorimetric, and spectroscopic techniques. They combined the resulting measurements with complex calculations. Their results show how molecules move, bind, form, and release in both pathways and the amount of energy involved. "We would not have succeeded without using this integrated approach," said Shi.
They performed experiments with two key techniques. Infrared spectroscopy provides details by exploiting how molecules absorb light in the infrared region. Thermogravimetry-differential scanning calorimetry characterizes the physical and chemical properties of the molecules as a function of increasing temperature. The team designed experiments with a significantly wider range of conditions, including higher pressures, compared to literature precedents. In the experiments, they used a clean sample that lacked interfering structural factors.
Their results established the relative population, as well as the energetics, of the key surface intermediates under the reaction conditions. For the monomer pathway, one propanol molecule enters the catalyst's active site. It binds to the active site, picking up a proton. Water is broken off, but it enables an intermediate to form. It leads to a nonsymmetrical molecule that's got a proton bridge to the catalyst. The catalyst's active site is inoperative until the intermediate is untethered, forming the desired propene.
For the dimer pathway, two propanol molecules enter the active site. This leads to a charged two-part, or dimer, species. This dimer is very stable and energy must be added to cleave the right bonds to form an intermediate that returns protons to the catalyst and releases both water and propanol. This intermediate is different from that created in the monomer path. The energy needs are also different.
"The team did not cut corners by assuming that conclusions reached in one catalytic system would necessarily hold true for another," said Camaioni.
In this study, the water was in the gas phase. In the next study, the team will be working with liquid water rather than steam. This research is part of an ongoing program, funded by the Office of Science at the U.S. Department of Energy. The program's goal is to overcome the fundamental questions that are holding us back from designing faster, cheaper, more efficient ways to create biofuels.
More information: Yuchun Zhi et al. Dehydration Pathways of 1-Propanol on HZSM-5 in the Presence and Absence of Water, Journal of the American Chemical Society (2015). DOI: 10.1021/jacs.5b09107
Journal information: Journal of the American Chemical Society
Provided by Pacific Northwest National Laboratory