Simple computer models unravel genetic stress reactions in cells
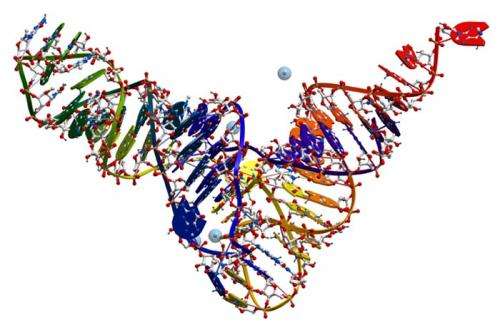
(Phys.org)—Many questions arise when two identical twins raised in the same home—fed the same, nurtured the same way—follow disparate paths. How can the identical offspring of a single egg turn out so differently?
Scientists ask the same question when genetically identical cells in identical environments—monoclonal cells produced by a single ancestor that replicated—exhibit wildly different behaviors. Researchers say the changes may be due to random biochemical fluctuations known as stochasticity, or "noise"—variability occurring over time and influenced by environmental factors.
Cells are highly sensitive to this noise, and minor fluctuations can lead to major changes, such as an Alzheimer's gene turning on or off or a cancer cell not responding to chemotheraphy. Are these random events due to chance or is there an undiscovered cause?
The answer might be contained within nearly undetectable processes—the noise—at the molecular level, so researchers must peer into individual cells really, really closely without nudging and accidentally altering these sensitive cells and their processes. This is complicated in itself, there are so many cells—about 100 trillion in each human. All that can get lost in the noise, and even supercomputers cannot hope to capture all aspects of this incredible complexity.
But experimental and computational scientists developed methods to sift through all the complexity and improve the illusive process of prediction with a simple model.
Unraveling genetic stress reactions
Brian Munsky, a scientist at Los Alamos National Laboratory's Center for Nonlinear Studies, is adept at untangling biology. Munsky and colleagues report their combined experimental and modeling prediction methods in the Feb. 1, 2013 edition of Science. These methods integrate single-cell experiments and discrete stochastic analysis to predict complex gene expression and signaling behaviors in Saccharomyces cerevisiae—or yeast, a scientific-lab standard since yeast and human cells share many genes. Scientists frequently test drugs or biological processes on yeast before advancing to human trials.
Using a technique called smFISH (single-molecule fluorescence in situ hybridization), the team attached dozens of small fluorescently labeled probes to each molecule of specific messenger ribonucleic acid (RNA), which causes these molecules to light up under the microscope. Some cells turn on (lots of lights) while others remain off. This data was incorporated into several models. Munsky notes that it would be impossible to combine the infinite possible reactions at different scales into a single computational model.
The combined, data-driven approach that includes validation allows researchers to systematically determine when models are too simple, too complex or just right—the "Goldilocks" approach.
The approach developed in this study helps researchers understand cellular death at the single-molecule and single-cell level—with wide applications
The research team identified predictive models of transcription—the first step in gene expression—when the yeast cell is responding to osmotic stress (salt), which greatly affects cell growth. Understanding how yeast cope with osmotic stress is useful to understand how human cells respond to medical treatments, but the principles are also applicable to many other fields, including agriculture.
"Depending upon molecular fluctuations, a single gene in a cell may switch randomly between active and inactive states, leading to vastly different biological responses, even for genetically identical cells," says Munsky. "The fluctuations can be quantified at the single-molecule and single-cell levels."
Munsky was a joint first author on the Science paper with Vanderbilt University's Gregor Neuert. The paper was selected as an Editors' Choice by the magazine.
"The approach we developed in this study is applicable far beyond yeast," Munsky adds. "Our experimental and computational analyses could enable quantitative prediction for any gene, pathway or organism."
More information: Read the Science article: "Systematic Identification of Signal-Activated Stochastic Gene Regulation"
Journal information: Science
Provided by Los Alamos National Laboratory