June 11, 2010 feature
For the first time, researchers observe graphene sheets becoming buckyballs (w/ Video)
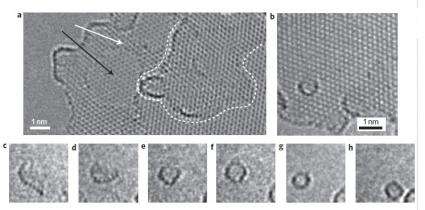
(PhysOrg.com) -- Peering through a transmission electron microscope (TEM), researchers from Germany, Spain, and the UK have observed graphene sheets transforming into spherical fullerenes, better known as buckyballs, for the first time. The experiment could shed light on the process of how fullerenes are formed, which has so far remained mysterious on the atomic scale.
“This is the first time that anyone has directly observed the mechanism of fullerene formation,” Andrei Khlobystov of the University of Nottingham told PhysOrg.com. “Shortly after the discovery of fullerene (exactly 25 years ago), the ‘top down’ mechanism of fullerene assembly was proposed. However, it was soon rejected in favor of a multitude of different ‘bottom up’ mechanisms, mainly because people could not understand how a flake of graphene could form a fullerene and because they did not have means to observe the fullerene formation in situ.”
As the scientists report in a recent study published in Nature Chemistry, there are four main steps involved in this top-down fullerene formation process, which can be explained by quantum chemical modeling. The critical first step is the loss of carbon atoms at the edge of the graphene sheet. Because the carbon atoms at the edge of graphene are connected by only two bonds to the rest of the structure, the researchers could use the microscope’s high-energy electron beam (or “e-beam”) to chip the atoms away, one by one. While exposed to the e-beam, the edges of the graphene sheet appear to be continuously changing shape.
The loss of carbon atoms on the edge of the graphene is the most crucial step in the process, the scientists explain, since it destabilizes the structure and triggers the subsequent three steps. The increase in the number of dangling carbon bonds at the edge of the graphene causes the formation of pentagons on the graphene edge, which is followed by the curving of the graphene into a bowl-like shape. Both of these processes are thermodynamically favorable, since they bring carbon atoms on the edge closer to one another, allowing them to form bonds with each other.
In the fourth and final step, the carbon bonds cause the curved graphene to “zip up” its open edges and form a cage-like buckyball. Because the zipping process reduces the number of dangling bonds, the spherical fullerene represents the most stable configuration of carbon atoms under these conditions. Once the edges are completely sealed, no further carbon atoms can be lost, and the newly created fullerene remains intact under the e-beam.
Although spherical fullerenes can already be generated in high yields from graphite (which is made of many graphene sheets stacked together), up until now scientists have not fully understood the underlying mechanisms of their formation. By observing the process in real time in this study, the researchers have been able to identify the structural changes that the graphene undergoes in order to become increasingly round and form a perfect fullerene. The results help to unravel the mystery of fullerene formation by explaining, for instance, how laser ablation works as a fullerene production method: the microscope’s e-beam, similar to a laser beam, supplies the energy to break the carbon bonds and serve as the critical initial step in the formation process.
“The key to the direct visualization of fullerene formation is (i) atomically thin graphene flakes mounted perpendicular to the electron beam; (ii) aberration corrected high resolution TEM allowing imaging with atomic resolution; and (iii) careful analysis of the evolution of graphene to fullerene, image simulation and correlation of the experimental data with theoretical calculations,” Khlobystov said. “This is why our study discovers so much more than previous TEM studies.”
In addition, the results help explain the high abundance of C60 and C70 fullerenes (fullerenes composed of 60 or 70 carbon atoms) found in different methods of fullerene production. The researchers found that a large (more than 100 carbon atoms) initial graphene flake imposes a significant energy penalty during the curving step, so that its edges continue to be chipped away until it is small enough to curve. On the other hand, very small (less than 60 atoms) graphene flakes experience excessive strain on the carbon bonds during the curving step, preventing them from closing up. So to enable the thermodynamically driven formation process, fullerenes end up having a narrow range of diameters averaging about one nanometer, which corresponds to 60-100 carbon atoms.
“Understanding the fullerene formation process teaches us about the fundamental connection between different forms of carbon,” Khlobystov said. “Also, it opens new avenues for fabrication of molecular nanostructures using the e-beam. This is a new way to do chemistry and to study molecules!”
More information: Andrey Chuvilin, et al. “Direct transformation of graphene to fullerene.” Nature Chemistry, Vol. 2, June 2010. DOI: 10.1038/NCHEM.644
Copyright 2010 PhysOrg.com.
All rights reserved. This material may not be published, broadcast, rewritten or redistributed in whole or part without the express written permission of PhysOrg.com.