This article has been reviewed according to Science X's editorial process and policies. Editors have highlighted the following attributes while ensuring the content's credibility:
fact-checked
peer-reviewed publication
trusted source
proofread
A new mathematical 'blueprint' is accelerating fusion device development
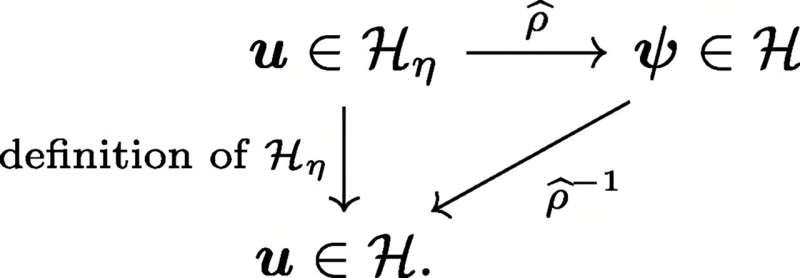
Developing commercial fusion energy requires scientists to understand sustained processes that have never before existed on Earth. But with so many unknowns, how do we make sure we're designing a device that can successfully harness fusion power?
We can fill gaps in our understanding using computational tools like algorithms and data simulations to knit together experimental data and theory, which allows us to optimize fusion device designs before they're built, saving much time and resources.
Currently, classical supercomputers are used to run simulations of plasma physics and fusion energy scenarios, but to address the many design and operating challenges that still remain, more powerful computers are a necessity, and of great interest to plasma researchers and physicists.
Quantum computers' exponentially faster computing speeds have offered plasma and fusion scientists the tantalizing possibility of vastly accelerated fusion device development. Quantum computers could reconcile a fusion device's many design parameters—for example, vessel shape, magnet spacing, and component placement—at a greater level of detail, while also completing the tasks faster. However, upgrading to a quantum computer is no simple task.
In a paper, "Dyson maps and unitary evolution for Maxwell equations in tensor dielectric media," recently published in Physics Review A, Abhay K. Ram, a research scientist at the MIT Plasma Science and Fusion Center (PSFC), and his co-authors Efstratios Koukoutsis, Kyriakos Hizanidis, and George Vahala present a framework that would facilitate the use of quantum computers to study electromagnetic waves in plasma and its manipulation in magnetic confinement fusion devices.
Quantum computers excel at simulating quantum physics phenomena, but many topics in plasma physics are predicated on the classical physics model. A plasma (which is the "dielectric media" referenced in the paper's title) consists of many particles—electrons and ions—the collective behaviors of which are effectively described using classic statistical physics. In contrast, quantum effects that influence atomic and subatomic scales are averaged out in classical plasma physics.
Furthermore, the descriptive limitations of quantum mechanics aren't suited to plasma. In a fusion device, plasmas are heated and manipulated using electromagnetic waves, which are one of the most important and ubiquitous occurrences in the universe.
The behaviors of electromagnetic waves, including how waves are formed and interact with their surroundings, are described by Maxwell's equations—a foundational component of classical plasma physics, and of general physics as well. The standard form of Maxwell's equations is not expressed in "quantum terms," however, so implementing the equations on a quantum computer is like fitting a square peg in a round hole: it doesn't work.
Consequently, for plasma physicists to take advantage of quantum computing's power for solving problems, classical physics must be translated into the language of quantum mechanics. The researchers tackled this translational challenge, and in their paper, they reveal that a Dyson map can bridge the translational divide between classical physics and quantum mechanics.
Maps are mathematical functions that demonstrate how to take an input from one kind of space and transform it to an output that is meaningful in a different kind of space. In the case of Maxwell's equations, a Dyson map allows classical electromagnetic waves to be studied in the space utilized by quantum computers. In essence, it reconfigures the square peg so it will fit into the round hole without compromising any physics.
The work also gives a blueprint of a quantum circuit encoded with equations expressed in quantum bits ("qubits") rather than classical bits so the equations may be used on quantum computers. Most importantly, these blueprints can be coded and tested on classical computers.
"For years we have been studying wave phenomena in plasma physics and fusion energy science using classical techniques. Quantum computing and quantum information science is challenging us to step out of our comfort zone, thereby ensuring that I have not 'become comfortably numb,'" says Ram, quoting a Pink Floyd song.
The paper's Dyson map and circuits have put quantum computing power within reach, fast-tracking an improved understanding of plasmas and electromagnetic waves, and putting us that much closer to the ideal fusion device design.
More information: Efstratios Koukoutsis et al, Dyson maps and unitary evolution for Maxwell equations in tensor dielectric media, Physical Review A (2023). DOI: 10.1103/PhysRevA.107.042215
Journal information: Physical Review A
Provided by Massachusetts Institute of Technology
This story is republished courtesy of MIT News (web.mit.edu/newsoffice/), a popular site that covers news about MIT research, innovation and teaching.