Redesigning lithium-ion battery anodes for better performance
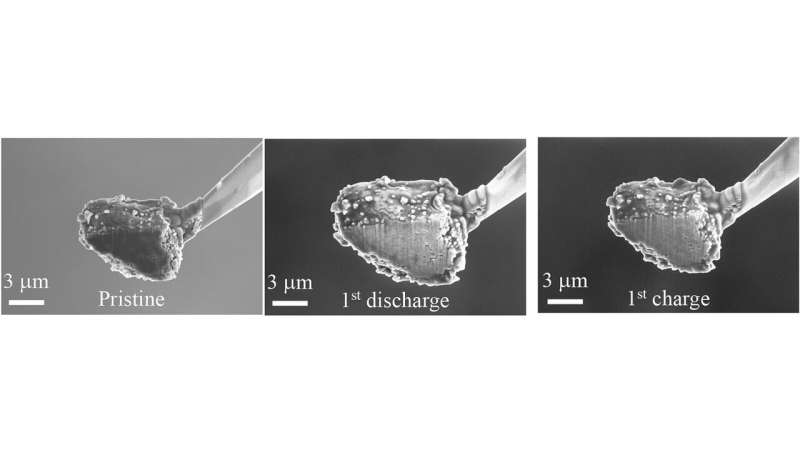
Scientists at Argonne National Laboratory have made progress toward a higher-capacity lithium-ion battery to meet rising consumer demand.
With the growing number of electric vehicles on the road and an increasing reliance on consumer electronics, demand has never been greater for development of lithium-ion batteries (LIBs) that can sustain a higher energy capacity, or amount of charge stored within the battery.
One way to increase the overall energy capacity of LIBs is to increase the energy capacity of the anode, or the negative electrode. For the past several decades, state-of-the-art LIBs have been made with graphite anodes. Graphite's energy capacity is stable, meaning the capacity does not fade, and the material does not crack even after more than 1000 full charge-discharge cycles. However, graphite has a low theoretical energy capacity, which cannot meet the increasing energy demands of today's society.
In a new study, a team led by researchers at the U.S. Department of Energy's (DOE) Argonne National Laboratory have demonstrated the increased capability of a potential new, higher-capacity anode material. This composite material had originally been developed for sodium-ion batteries, which are more infrequently commercially used than lithium-ion batteries. This new study sought to apply the material to lithium-ion batteries.
Recently, two materials have been at the forefront of research for next-generation battery anodes—silicon and phosphorus. Both silicon and phosphorus have a theoretical energy capacity at least 10 times greater than graphite, meaning they could surpass the energy capacity requirements for LIBs. According to senior materials scientist and Argonne Distinguished Fellow Khalil Amine, the lead researcher of the Argonne study, silicon has two major issues. The first issue involves the high-volume expansion when silicon is lithiated during charging, which would likely cause the anode material to break apart. Cracking would lead to a loss of energy capacity, he explained.
The second issue involves a term called initial coulombic efficiency (ICE). When a battery goes through a full charge-discharge cycle, the charge output of the battery theoretically should match the charge input. However, some energy in the charge output is lost to the lithium reacting with the anode material. To develop a practical LIB, the ratio of the charge output compared to the charge input on the first charge-discharge cycle should be above 90%. This ratio is the ICE. With silicon, the ICE is less than 80%, which Amine explained renders it infeasible for practical use.
In their research, Amine, Argonne chemist Gui-Liang Xu, and their colleagues explored two potential types of phosphorus: black and red phosphorus. "Phosphorus has a very high energy capacity," Xu said. "When we explored the material, we found that our anode material has a very high ICE of more than 90%."
An ICE of more than 90% demonstrates that very few side reactions occur between the anode material and the electrolyte, so not much lithium is lost during the initial charging and discharging.
The team created their own anode composite composed primarily of black phosphorus—a highly conductive form of phosphorus with a high theoretical capacity—and conductive carbon compounds.
To create the composite, the researchers ground the bulk phosphorus material and conductive carbon into micrometer-sized particles, which increases the density of the anode.
When measuring the life cycles, or the total number of times a battery can be charged and discharged, Amine and his colleagues turned to Argonne's Advanced Photon Source (APS) and Center for Nanoscale Materials (CNM), both DOE Office of Science User Facilities. Employing in-situ storage ring light source X-ray diffraction at the APS and in-situ scanning electron microscopy at the CNM, the team observed the anode's phase and volume transformation during repeated charging and discharging.
"Argonne has unique abilities available at the APS and CNM," Xu said. "With the storage ring light source, we can probe the phase transformation during lithiation and delithiation, which allows us to see the reaction reversibility."
After showing the stability of the black phosphorous composite, the team investigated a composite with red phosphorus instead of black phosphorus. Black phosphorus, though significantly more conductive than red phosphorus, is too expensive for practical use in the market. With the red phosphorus composite, which is an economically viable option, the battery showed a similar stability and high ICE, with a very high practical capacity.
The team is currently working on a composite material made mostly of red phosphorus, and the material shows promising results, Xu said. "We're trying to initiate collaboration with industry partners so we can scale up this material, so it can be commercialized in the future."
The research paper on the study, "A practical phosphorus-based anode material for high-energy lithium-ion batteries," appeared online on April 26, 2020, in Nano Energy.
More information: Rachid Amine et al. A practical phosphorus-based anode material for high-energy lithium-ion batteries, Nano Energy (2020). DOI: 10.1016/j.nanoen.2020.104849
Journal information: Nano Energy
Provided by Argonne National Laboratory