September 16, 2019 feature
Gravity surveys using a mobile atom interferometer
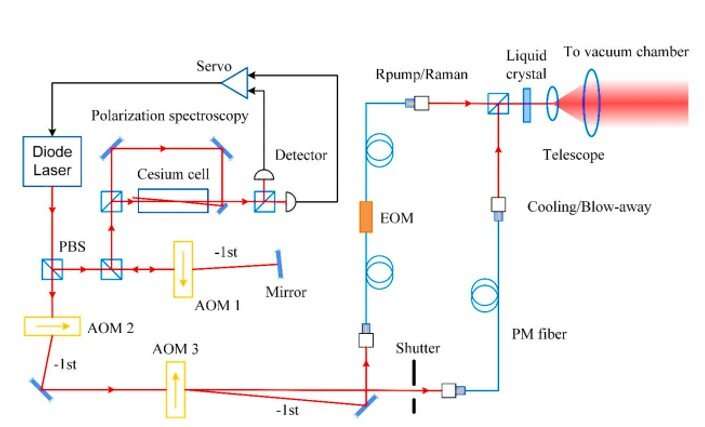
Mobile gravimetry is an important technique in metrology, navigation, geodesy and geophysics. Although atomic gravimeters are presently used for accuracy, they are constrained by instrumental fragility and complexity. In a new study, Xuejian Wu and an interdisciplinary research team in the departments of physics, the U.S. Geological Survey, molecular biophysics and integrated bio-imaging, demonstrated a mobile atomic gravimeter. The device measured tidal gravity variations in the lab and surveyed gravity in the field.
They used the equipment to achieve a high sensitivity for tidal gravity measurements with long-term stability to reveal ocean tidal loading effects, as well as several distant earthquakes. The research team surveyed gravity in the Berkeley Hills to determine the density of subsurface rocks from the vertical gravity gradient. The simple and sensitive instrument developed in the study will pave the way to bring atomic gravimeters to field applications. The work is now published on Science Advances.
Physicists typically use light-pulse atom interferometers to measure inertial forces alongside studies to understand sub-gravitational forces on atoms. Gravimeters based on atom interferometry are among the most accurate and sensitive tools to precisely measure gravity, in contrast to existing instruments based on springs, superconducting coils, micromechanical devices or falling corner cubes. Atomic gravimeters rely on matter-wave interferometry measurements with a freely falling atomic cloud. In its mechanism of action, scientists can steer matter waves into two interferometer arms using the momentum of photons that are extremely well defined by the integrated laser wavelength.
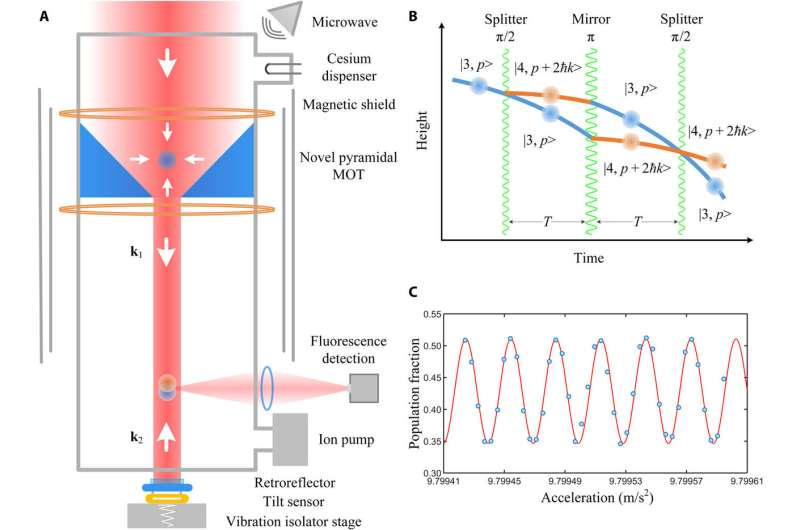
Researchers are presently engineering transportable atomic gravimeters for applications in metrology, airborne sensing, shipborne surveys and field applications. Such instruments typically reach sensitivities around 5 to 100 µGalileo (µGal) in the lab, while the atomic gravimeter in gravity surveys had only achieved a precision approximating 1 mGal on a marine ship. Precise mobile gravimetry is therefore valuable for gravity measurements with an uncertainty of a few microGalileos in metrology. For instance, to aid inertial marine navigation, gravity reference maps require gravimeters with at least miliGalileo accuracy on board. As a result, atomic gravimeters should be both sensitive and mobile for reliable applications in the field.
In the present work, Wu et al. demonstrated a mobile atomic gravimeter in the lab and during field operations. The research team compared gravity measured in the experiments with a solid Earth tide model to indicate its atomic sensitivity. Based on the instrumental sensitivity Wu et al. observed ocean tide loading effects and measured seismic waves of distant earthquakes. The research team then implemented gravity surveys in Berkeley Hills using the instrument. The atomic gravimeter can be used for geodetic and geophysical studies to refine the geoid during resource exploration, hydrological studies and hazard monitoring for precise field measurements in the future.
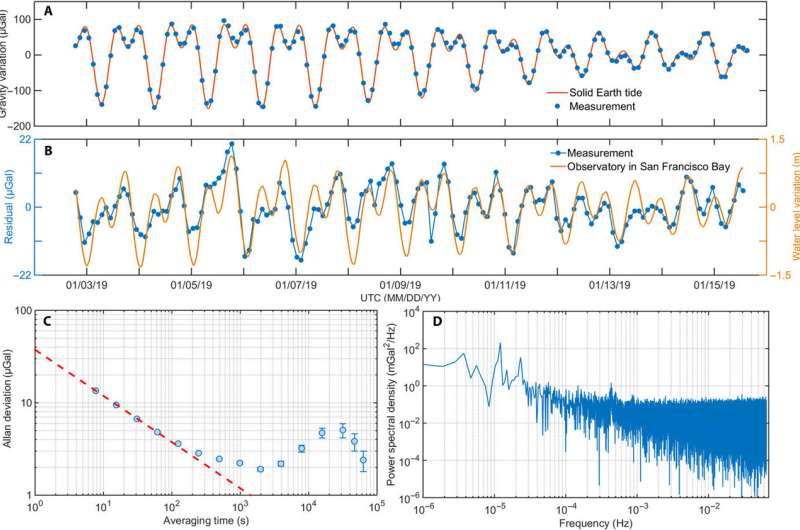
Wu et al. engineered the mobile atomic gravimeter on an atom interferometer featuring a magneto-optical trap (MOT) inside a pyramid mirror with a through-hole. This novel geometry offered many advantages; by first forming a differential pumping stage between the MOT and atom interferometry regions, with a vapor pressure ratio of more than 10:1 to accelerate atom-loading speed and decreased background noise for atom detection. The setup allowed the MOT and interferometer laser beams to have different waists to achieve a large MOT volume and high Raman beam intensity with available laser power. As a third feature, the research team enabled the atomic gravimeter to take advantage of retroreflection from a vibration-isolated mirror insensitive to vibrations of the pyramid mirror. The vibration isolation was simpler and effective compared to traditional pyramidal atomic gravimeters. For its fourth feature, Wu et al. used a flat mirror as the retroreflector to eliminate systematic effects from imperfections in the pyramidal setup.
The team performed atom interferometry underneath the pyramid mirror using Doppler-sensitive two-photon Raman transitions driven by two laser beams and a Mach-Zehnder geometry. Since the atoms moved in free fall, the scientists ramped the laser frequency difference between the two beams with a rate of α, which they varied to obtain acceleration in the system. They used a single diode laser with three acousto-optic modulators (AOMs) and one fiber-based electro-optic phase modulator (EOM), to generate all laser beams necessary for the MOT, during interferometry and detection procedures of the study.
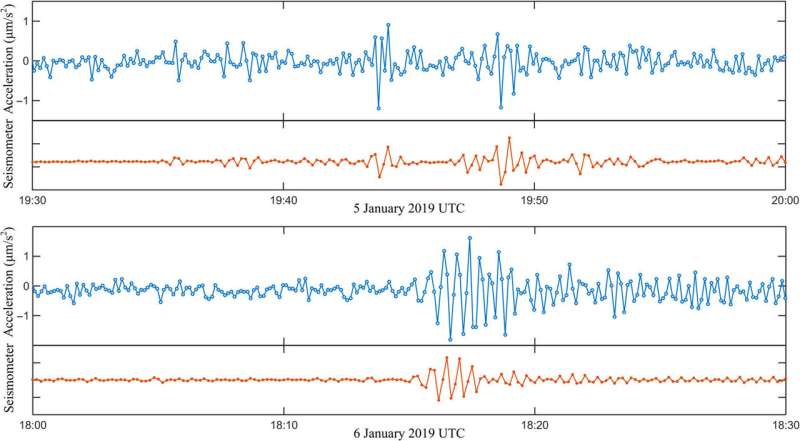
The scientists measured long-term tidal gravity variation for 12 days using the atomic gravimeter. They then operated the atom interferometer with active vibration isolation and obtained mean values of gravity data every 2 hours, compared with a solid Earth tide model. Since the research lab was located about 4.5 km east of San Francisco Bay area, the ocean tidal loading effect on gravity was notable at the precise location in contrast to previous reports. The researchers corrected for the solid Earth tide and obtained a sensitivity of 37 µGal/√Hz for the atomic gravimeter with stability greater than 2 µGal within half an hour. During tidal gravity measurements, the atomic gravimeter could record seismic wave trains from several distant earthquakes to measure vertical acceleration of the seismic waves. Wu et al. compared the atomic gravimeter with one of the seismometers in the Berkeley Digital Seismic Network. For instance, when a 6.8-magnitude and 570 km deep earthquake occurred in Brazil on January 5, 2019, both the atomic gravimeter and seismometer detected body waves from the earthquake after about 20 mins. The team in Berkeley similarly detected measurements on January 6, 2019, when a 6.6-magnitude and 43-km deep earthquake occurred in Indonesia.
To investigate the accuracy of the atomic gravimeter, the research team estimated systematic effects. They calculated the total systematic error at 0.015 mGal with a measurement bias approximating -0.008 mGal. The researchers verified the repeatability of the experiment in-house after transporting the atomic gravimeter to the Campbell Hall at the University of California Berkeley Campus, to measure gravity on different floors, with gravity on the basement floor as a reference. The values matched those calculated using standard gravity survey techniques. Depending on the vibrational noise, the atomic gravimeter achieved a sensitivity of around 0.2 mGal/√Hz. However, the sensitivity on higher floors decreased due to stronger vibrations. The results indicated the gravitational effect of the mass of the Campbell building.
![Gravity survey in Berkeley Hills. (A) Measurement route. The blue curve depicts the route, and the white pin drops are the six measurement locations. (B) Gravity anomaly as a function of the elevation. Elevations are from Google maps. The error bars are 1 − σ statistical and systematic errors. The dashed line indicates a VGG of −0.225(10) mGal/m. (C) The atomic gravimeter apparatus. (D) Field operation of the atomic gravimeter inside a vehicle. [Photo credit for (A): Google Maps; photo credit for (C) and (D): Xuejian Wu, UC Berkeley]. Credit: Science Advances, doi: 10.1126/sciadv.aax0800. Gravity surveys using a mobile atom interferometer](https://scx1.b-cdn.net/csz/news/800a/2019/4-gravitysurve.jpg)
Thereafter, the team used the atomic gravimeter in the field to survey absolute gravity in the Berkeley Hills. They operated the gravimeter inside a vehicle on a route length of 7.6 km and an elevation change of 400 m, while using passive vibration isolation to measure gravity in 6 locations. The team spent approximately 15 mins to setup the gravimeter at each location, which included pairing up the instrument and aligning the interferometer beam to the gravity axis. Due to increased vibrational noise in the field, Wu et al. measured the gravimeter sensitivity at 0.5 mGal//√Hz. In total, the measurements showed approximate gravity changes by 92.6 mGal, from the base to the peak of the Berkeley Hills.
In this way, Xuejian Wu and colleagues developed a mobile atomic gravimeter to perform tidal gravity measurements and gravity surveys. The novel pyramidal MOT instrument took advantage of single-beam atom interferometry to offer simple laser-to-gravity alignment and enhanced vibration isolation. The device is mobile, compact and robust for transportation in the field, while maintaining comparatively higher sensitivity to the existing atomic gravimeters. The features allow geodetic and geophysical applications for precise mobile gravimetry in the lab and on the field. The instrument is presently limited by vibrational noise with room for improvement. Advanced gravimeters will find additional applications as tunnel detectors, sensors for underground water storage and monitor earthquakes and volcanic activity.
More information: Xuejian Wu et al. Gravity surveys using a mobile atom interferometer, Science Advances (2019). DOI: 10.1126/sciadv.aax0800
G. Rosi et al. Precision measurement of the Newtonian gravitational constant using cold atoms, Nature (2014). DOI: 10.1038/nature13433
Matt Jaffe et al. Testing sub-gravitational forces on atoms from a miniature in-vacuum source mass, Nature Physics (2017). DOI: 10.1038/nphys4189
Journal information: Science Advances , Nature , Nature Physics
© 2019 Science X Network