Gravitational waves found again: here's how they could whisper the universe's secrets
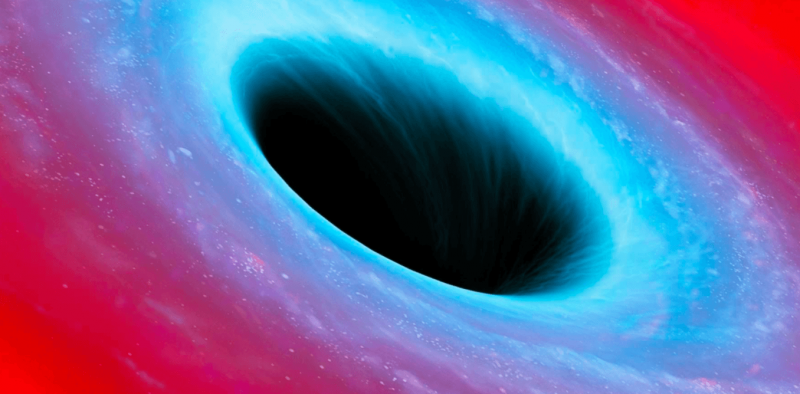
The international team of physicists and astronomers responsible for the discovery of gravitational waves back in February has announced the detection of a second strong signal from the depths of space. It is further confirmation that gravitational waves both exist and tell us a whole new story about how the universe came to be the way it is.
In 1915 Albert Einstein put forward his general theory of relativity, making the bold step of equating gravity to distortions of time and space caused by the presence of mass. He and others went on to predict that accelerating masses would generate ripples of distortion, flowing out as gravitational waves, though it was thought these would be too weak to ever measure.
Like the first detection, this second fleeting signal came from two black holes in a tight orbit – what's known as a binary black hole system. After spending many millions of years orbiting each other in ever decreasing circles, these black holes collided in a fraction of a second over a billion years ago.
They had total mass around three times smaller than in the first detection – roughly 22 times the mass of our sun – but once again the collision shook the structure of the universe so violently that we detected the waves of stretched and squeezed space and time as they passed through us at the speed of light on Boxing Day last year.
Even so, this did not produce the kind of clearly visible waveform that heralded the first detection. It only stood out strongly once processed by the software the team developed over many years to extract the maximum amount of science from our three gravitational wave detectors – the two that comprise Advanced LIGO (Laser Interferometer Gravitational Wave Observatory) in the US and Advanced Virgo in Italy.
Questions and possible answers
The existence of two black holes with such high masses came as a surprise to many astronomers at the time of the first announcement, since the formation routes for such black holes are unclear. It was in fact the first direct evidence that binary black holes exist and can collide and merge.
The existence of massive black holes raises many questions: were they formed in the Big Bang or through the collapse of matter at a later date? Did they form from a pair of supermassive stars or did they join together by chance once the stars had become black holes? Are they related to the formation of the supermassive black holes that appear to exist in the cores of nearly all galaxies? And what are the implications for the collapse of matter into stars and galaxies and the formation of structure in the universe?
When it comes to answering questions such as these, the number of events we see is important. Everything we have seen to date was the result of the inaugural four-month run of Advanced LIGO – two spectacular deaths of binary black hole systems (and possibly also a third one, though the signal is not strong enough for us to say with certainty).
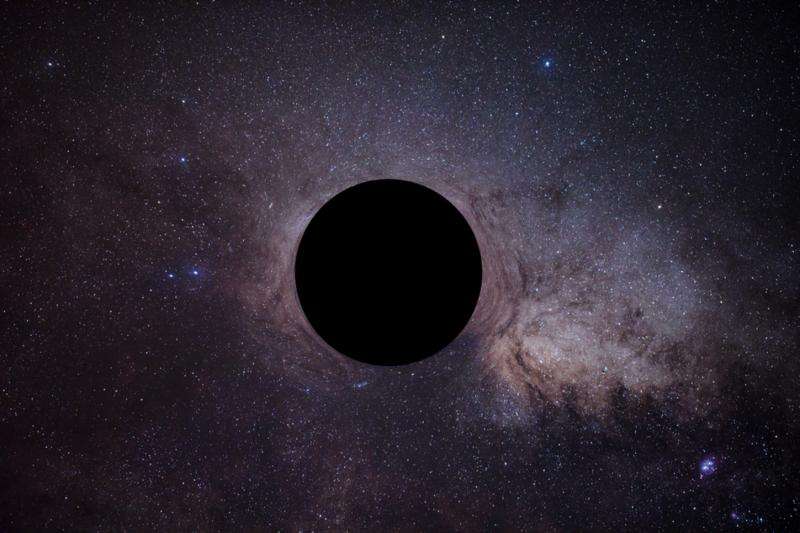
The tantalising implication is that we will see many more in future. This would let us see the full range of masses of binary black holes, and also how rapidly they spin and how their spin axes are aligned. These are crucial factors in determining how black holes are formed.
As if that wasn't enough, these signals give us something else. It's basic, but in astronomy it is a very highly prized number: the distance to their source. There are remarkably few reliable ways to measure the biggest distances in the universe and gravitational astronomy provides one that is entirely new. As the field advances and new telescopes become available, this could help us map the expansion of the universe and examine the elusive ideas of "dark matter" and "dark energy" that run through current cosmological theories.
When the Advanced LIGO detectors return to operation towards the end of the year it will be with even greater sensitivity and they will observe for longer, this time in conjunction with Advanced Virgo. As a result we can reasonably hope to see approximately ten further binary black hole signals by this time next year. By 2018 we predict that we will see many tens, maybe even hundreds of such signals, allowing us to study the population of these enigmatic sources more deeply.
The improved sensitivity and the third detector in Italy also open the door to even more exciting discoveries to come. We are hoping to observe gravitational waves from neutron stars. These are the remnants of lower-mass stars whose lower gravity prevented them from collapsing all the way to black holes. Unlike a black hole, a neutron star is a highly compressed ball of nuclear matter and its detailed structure is set by particle physics.
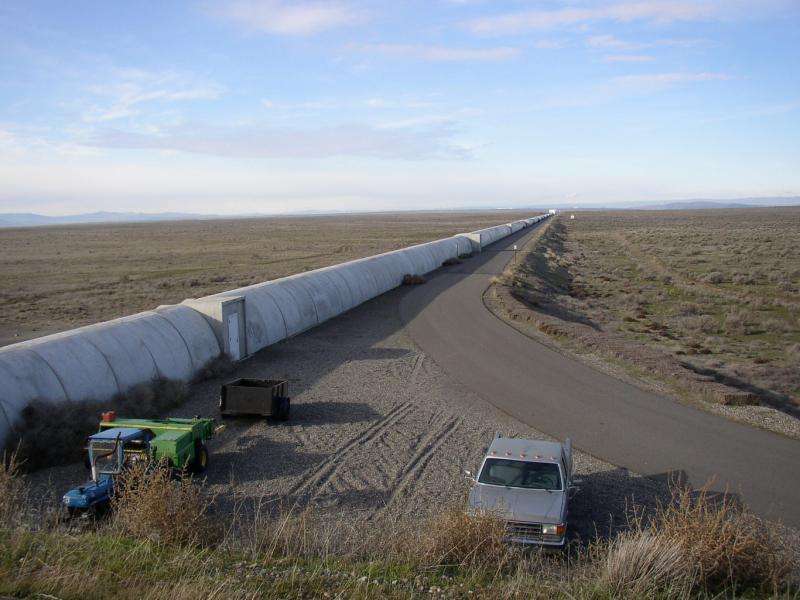
Observing gravitational waves either from single neutron stars or binary pairs would reveal the physics of matter under the most extreme conditions in the universe. Having three detectors working in conjunction will also mean that we can be more accurate about the position in the sky from which a wave is coming. That will help us to identify any signals from these sources seen by conventional telescopes – the flash from the collision or its aftermath. This will multiply the scientific return from both kinds of signals many times over, for example by giving us more insight into the structure of the universe.
Looking ahead a few years, we will have space-based gravitational wave observatories of the kind pioneered by the recent highly successful LISA Pathfinder Mission (which announced its own astonishing performance results earlier in June). This would allow us to see a new spectrum of sources at lower frequencies, but could also reveal these black hole binaries earlier in their lives, and allow us to follow them for years before they finally coalesce. In short, the spectacular results from this first short run of LIGO are just the beginning for gravitational astronomy.
Source: The Conversation
This article was originally published on The Conversation. Read the original article.