February 8, 2016 feature
Could 80-year-old ether experiments have detected a cosmological temperature gradient?
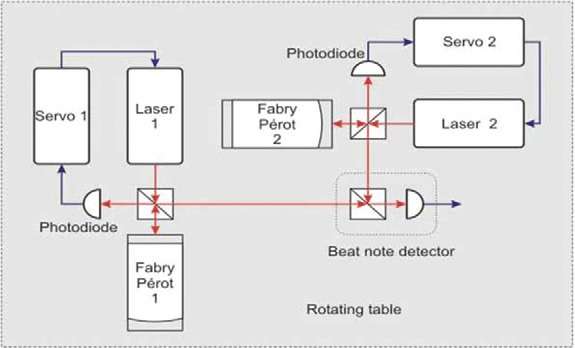
(Phys.org)—In a new study, scientists have proposed that tiny residual effects measured by ether-drift experiments in the 1920s and '30s may be the first evidence of a temperature gradient that was theorized in the 1970s, but never before detected in a laboratory. The theorized temperature gradient is thought to be caused by the solar system moving at 370 km/sec through the cosmic background radiation (CBR), which is the faint electromagnetic radiation that fills the universe.
The researchers, Maurizio Consoli from INFN, along with Alessandro Pluchino and Andrea Rapisarda from INFN and the University of Catania in Italy, have published a paper on their reinterpretation of the ether-drift experiments in a recent issue of EPL.
"The main significance of our work is that those small residual effects found in all ether-drift experiments in gaseous systems, usually considered as uninteresting thermal disturbances, might instead represent the first experimental evidence for the Earth's motion within the CBR," Consoli told Phys.org.
"The interest of our work is twofold. On the one hand, there is an important historical interest because these small effects were obtained well before the discovery of the CBR (in 1965 by Penzias and Wilson, Astrophys. J. Lett. 142 (1965) 419) and well before the observation of the Earth's motion within the CBR (in 1977 by Smoot, Gorenstein and Muller, Phys. Rev. Lett. 39 (1977) 898, with the U2 experiment). On the other hand, the agreement between optical measurements in a laboratory and direct measurements of the CBR in space would indicate how powerful optical interferometers can be."
Background
In the 19th century, one of the most controversial questions in science was whether empty space was truly empty like a vacuum or whether it contained a medium known as ether. Ether had been proposed as a medium through which light waves could travel, since light's wave-like properties had recently been discovered, and it was believed that all waves needed some kind of medium to propagate through.
The first crucial experiment to provide evidence that ether does not exist as classically predicted came in 1887, when Michelson and Morley famously showed that light travels at the same speed in the perpendicular arms of an interferometer. If ether existed—and if it behaved as expected—it would be predicted to slow light down more in one direction than in the perpendicular direction, since motion through the ether is "anisotropic," or directionally dependent.
According to Consoli, Pluchino, and Rapisarda, however, the Michelson-Morley experiment did not yield a strictly null result because of its limited precision. The classically predicted effect of ether-drift was 10-8, while the Michelson-Morley experiment had a precision of 10-9—precise enough to rule out the classically predicted effect, but hazy enough to leave some room for the possibility of a smaller-than-expected effect.
In the years since, 20 or so similar experiments have been performed that further increased the precision, with the most recent test achieving the highest precision yet of 10-18 just last year (M. Nagel et al., Nature Comm. 6 (2015) 8174).
Scientists performing these tests today aren't as interested in disproving the existence of ether as they are in validating the foundations of Einstein's theory of special relativity. A difference in the speed of light in any two perpendicular directions in an inertial reference frame would contradict special relativity, since relativity requires a vacuum within which the speed of light is constant. Many important concepts emerge from this fact, including that the universe has no preferred reference frame, that there is no absolute space or time, and that you can never really tell for sure whether you are at rest or in constant motion since all motion is relative.
A matter of interpretation
At first glance, the 20 or so experiments performed since 1887 seem to have steadily improved the precision in support of the view that there is no ether and no preferred reference frame.
However, not all the results were perfectly unambiguous. In particular, Dayton Miller's 1933 optical interferometer experiment detected so-called "fringe shifts," which were much smaller than any expected effect but large enough to be considered non-negligible. To make things more complicated, these residual effects were irregular and not consistently reproducible.
At the time, the fringe shifts were written off to temperature differences inside the lab, since fluctuations of even a tiny fraction of a degree could have caused the observations. However, Miller disagreed with this interpretation. He argued that his measurements could not be explained by uniform heating, only by some non-uniform, directional effect.
Miller's experiment was not the only one to detect unexpectedly tiny effects. As Consoli and Pluchino, together with Caroline Matheson at the University of Cambridge, showed in a previous paper (Eur. Phys. J. Plus 128 (2013) 71 and arXiv:1302.3508 [physics.gen-ph]), all of the ether-drift experiments that were performed in gaseous media (either air or helium), including the original Michelson-Morley experiment, detected very small residual effects that were generally ignored.
In their new paper, the researchers argue that all of these residual effects may provide the first indirect evidence of the temperature anisotropy caused by the Earth's motion within the CBR. Previous direct observations of the CBR in space found that the entire solar system is moving at a velocity of about 370 km/sec toward a specific point in the sky, creating a "kinematic dipole," which is basically a Doppler effect. As a consequence, "an observer moving through the CBR would see different temperatures in different directions," as the researchers explain in their paper. But so far no study has actually measured in a laboratory the predicted temperature gradient caused by the solar system's movement.
The scientists explained that the temperature gradient is universal in the sense that all observers moving within the CBR (even hypothetical observers living on distant planets) will see a qualitatively similar effect. However, the quantitative aspects are different and depend on the particular state of motion of each planet and its surroundings.
"This motion is called 'peculiar' because it is characteristic of our local position in the universe," Pluchino said. "In fact, it is obtained by combining the motion of our galaxy, and of the local group of galaxies, with a velocity of about 600 km/sec toward what is called the Great Attractor (a large concentration of matter situated at about 100 Mpc from us), along with the motion of the solar system within our galaxy. Therefore, an observer placed at the opposite site of our galaxy will also see a dipole anisotropy but, for him, the kinematical parameters will be different."
By analyzing the data from the interferometer experiments in gaseous systems, the researchers found that all of the residual effects produced velocities in good agreement with the theoretical velocity of 370 km/sec.
"The average Miller fringe shift was giving exactly the same observable velocity as in Michelson-Morley," Pluchino said. "Therefore, the standard thermal interpretation of Miller's data is only acceptable if this temperature effect has a non-local origin, i.e., it does not depend on the particular conditions of its laboratory."
The scientists explain that the reason why this temperature effect and velocity had not been noticed before now is because their new interpretation is based on relativity.
"The basic difference with the standard point of view is that one should correctly reinterpret the observations according to relativity and not just use the (incorrect) classical formulas," Pluchino said.
The researchers also addressed the question of why not all of the ether-drift experiments detected fringe shifts, only those performed in gaseous media. As technology progressed over the decades, researchers carried out the experiments in different media, such as a vacuum or solid dielectrics. Traditionally, researchers have thought that the experiments were all testing the same thing, regardless of the medium.
But the Italian scientists think otherwise. If their proposal is correct, then the CBR temperature gradient would affect gaseous systems more strongly than others because the weakly bound gas molecules can be set in motion more easily by a thermal gradient than the molecules in a solid, which would not be expected to move very much. And in the case of a vacuum, a temperature gradient would have no matter to act on at all.
Implications
The scientists suggest that their interpretation could be tested by a new generation of precise laser interferometers, which like the early experiments could attempt to detect small differences in the speed of light between perpendicular directions in a gaseous medium. This type of experiment would provide a much more precise test than those of the '20s and '30s, and even more precise than the most recent test in a gaseous medium, which was performed in the '60s (which also detected residual time variations that the scientists show are consistent with the new interpretation).
If future experiments detect the expected signal, it would have far-reaching consequences on everything from physics to biology.
"If this temperature gradient could be definitely confirmed in a laboratory, it would mean that everything on Earth (and on any other celestial body moving through the CBR) is exposed to a tiny energy flow," Consoli said. "This flow is now very weak but, in the past, was substantially stronger when the CBR temperature was higher. Therefore, it has represented (and still represents) a sort of background noise which is independent of any localized source. It is known that such non-equilibrium condition can induce (or it could have induced) forms of self-organization in matter. Therefore, our result could also be relevant for those research areas which look for the origin of complexity in nature."
What do the results mean for special relativity?
"Clearly, the whole analysis uses a relativistic formalism," Consoli said. "But the final picture is different from standard special relativity. In fact, the temperature gradient due to the Earth's motion within the CBR can affect a weakly bound gaseous matter and, therefore, the velocity of light propagating inside it. Small differences in perpendicular directions can thus be detected with an interferometer. In this way, with measurements performed entirely inside a laboratory, one could distinguish between a state of rest and a state of uniform motion. This is not too surprising since the CBR was not known in 1905."
Rather than speculate on this area, the researchers prefer to focus on more practical implications.
"Our findings emphasize the power of optical interferometry in a laboratory," Consoli said. "This could give precious, complementary information to the direct observations of the CBR in space."
More information: M. Consoli, et al. "Cosmic Background Radiation and 'ether-drift' experiments." EPL. DOI: 10.1209/0295-5075/113/19001 . Also at arXiv:1601.06518 [astro-ph.CO]
Journal information: Europhysics Letters (EPL) , Physical Review Letters
© 2016 Phys.org