Controlling the thermodynamics of light
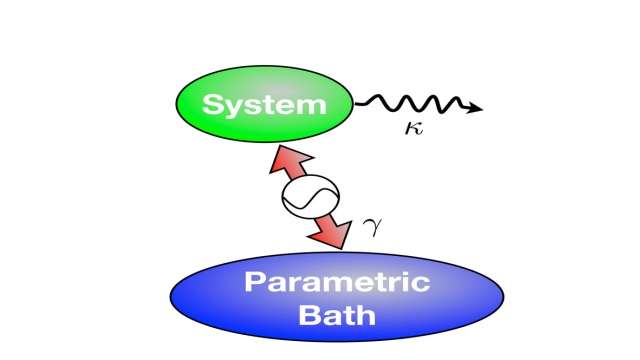
The concept of temperature is critical in describing many physical phenomena, such as the transition from one phase of matter to another. Turn the temperature knob and interesting things can happen. But other knobs might be just as important for some studying some phenomena. One such knob is chemical potential, a thermodynamic parameter first introduced in the nineteenth century scientists for keeping track of potential energy absorbed or emitted by a system during chemical reactions.
In these reactions different atomic species rearranged themselves into new configuration while conserving the overall inventory of atoms. That is, atoms could change their partners but the total number of identity of the atoms remained invariant.
Chemical potential is just one of many examples of how flows can be described. An imbalance in temperature results in a flow of energy. An imbalance in electrical potential results in a flow of charged particles. An imbalance in chemical potential results in a flow of particles; and specifically an imbalance in chemical potential for light would results in a flow of photons.
Can the concept of chemical light apply to light? At first the answer would seem to be no since particles of light, photons, are regularly absorbed when then they interact with regular matter. The number of photons present is not preserved. But recent experiments have shown that under special conditions photon number can be conserved, clearing the way for the use of chemical potential for light.
Now three JQI scientists offer a more generalized theoretical description of chemical potential (usually denoted by the Greek letter mu) for light and show how mu can be controlled and applied in a number of physics research areas.
A prominent experimental demonstration of chemical potential for light took place at the University of Bonn in 2010. It consisted of quanta of light (photons) bouncing back and forth inside a reflective cavity filled with dye molecules. The dye molecules, acting as a tunable energy bath (a parametric bath), would regularly absorb photons (seemingly ruling out the idea of photon number being conserved) but would re-emit the light. Gradually the light warmed the molecules and the molecules cooled the light until they were all at thermal equilibrium. This was the first time photons had been successfully "thermalized" in this way. Furthermore, at still colder temperatures the photons collapsed into a single quantum state; this was the first photonic Bose-Einstein condensate (BEC).
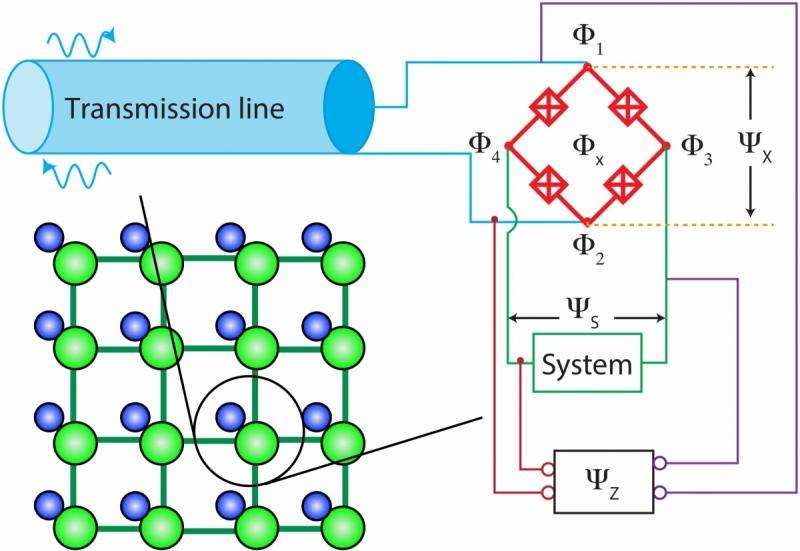
In a paper published in the journal Physical Review B the JQI theorists describe a generic approach to chemical potential for light. They illustrate their ideas by showing how a chemical-potential protocol can be implemented a microcircuit array. Instead of crisscrossing a single cavity, the photons are set loose in an array of microwave transmission lines. And instead of interacting with a bath of dye molecules, the photons here interact with a network of tuned circuits
"One likely benefit in using chemical potential as a controllable parameter will be carrying out quantum simulations of actual condensed-matter systems," said Jacob Taylor, one of the JQI theorists taking part in the new study. In what some call a prototype for future full-scale quantum computing, quantum simulations use tuned interactions in a small microcircuit setup to arrive at a numerical solution to calculations that (in their complexity) would defeat a normal digital computer.
In the scheme described above, for instance, the photons, carefully put in a superposition of spin states, could serve as qubits. The qubits can be programmed to perform special simulations. The circuits, including the transmission lines, act as the coupling mechanism whereby photons can be respectively up- or down-converted to lower or higher energy by obtaining energy from or giving energy to excitations of the circuits.
More information: M. Hafezi et al. Chemical potential for light by parametric coupling, Physical Review B (2015). DOI: 10.1103/PhysRevB.92.174305
Journal information: Physical Review B
Provided by Joint Quantum Institute