Accelerators and Light Sources of Tomorrow (Part 2: Accelerating with Light)
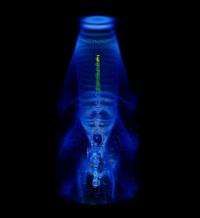
Accelerators are far from achieving the highest energies their builders aspire to, but size and cost may limit the kinds of facilities funding agencies can support. In the future, new kinds of machines will be needed to make further progress. Perhaps the most promising is the laser plasma accelerator.
Sophisticated as it is, a superconducting linac is a conventional particle accelerator that, in a machine like the Next Generation Light Source (NGLS) now under study, can be used to produce superbright laser beams. The inverse is also true: powerful lasers can be used to accelerate charged particles - but in ways that are anything but conventional.
An accelerator’s beam energy depends on the strength of its accelerating field (the gradient) and on its length. With gradients of 10 to 20 million volts per meter, the NGLS’s superconducting linac will continuously accelerate electrons to 2.5 billion electron volts (2.5 GeV) in a facility two-thirds of a kilometer long. That’s a short distance for a conventional accelerator, but when plasma accelerators come online sometime in the future, it may seem long indeed.
Laser plasma acceleration
Plasma accelerators were first proposed 30 years ago by the late John Dawson of the University of California at Los Angeles and Toshiki Tajima, now at the Ludwig Maximilian University of Munich. A plasma resembles an ordinary gas, one so hot that the atoms have fallen apart into their constituent particles - in the case of heated hydrogen gas, protons and electrons. To create a strong electric field it’s necessary to separate these positive and negative charges.
“Laser wakefield acceleration works on the principle that by sending a laser pulse through a gas to create a plasma — separating negatively charged electrons from positively charged ions in the gas — some of the free electrons will be carried along in the wake of the plasma wave created by the laser,” explains Wim Leemans, head of the LOASIS Program in Berkeley Lab’s Accelerator and Fusion Research Division. LOASIS stands for Laser Optics and Accelerator Systems Integrated Studies. “Imagine that the plasma is a lake and the laser pulse is a speedboat. The electrons are surfers riding the wave created by the boat’s wake.”
Theoretically, a laser wakefield accelerator could create an electric field of 100 billion volts per meter, 10,000 times as strong as conventional accelerators. Before the mid-1980s, however, lasers couldn’t make pulses short enough or powerful enough to achieve laser wakefield acceleration at all, because very high-powered lasers tended to blow themselves to pieces.
Then came a new laser technology called chirped-pulse amplification, invented by Gérard Mourou and Donna Strickland of the University of Rochester. The technique begins with a very short pulse from a very low-power oscillator. The short pulse is then “stretched” a thousand times or more with a pair of optical gratings that spread out the wavelengths, creating a “chirp” with low frequencies in the front of the pulse and high frequencies in back. The stretched-out chirp can be amplified a million times or more without damage to the laser. Once amplified, the pulse is unstretched with gratings or prisms and compacted back to its original short length and wide bandwidth - but now with ultrahigh power.
With chirped-pulse amplification laser wakefield acceleration advanced rapidly, although poor beam quality was a problem. Some electrons in a bunch achieved high energies but were tailed by many more electrons of low energy. “The energy spreads were so wide that they weren’t beams so much as sprays of electrons,” Leemans remarks.
But by 2004 three groups (one based in England, one in France, and the LOASIS team at Berkeley Lab) were closing in on the problem. They published their results simultaneously in Nature.
Two of the groups essentially used brute force, but the Berkeley Lab technique was unique, subtle, and promised a pathway to the future. To maintain beam focus, LOASIS created plasma channels that acted like optical fibers to concentrate and guide the laser pulse. An initial laser pulse drilled such a channel through a plume of hydrogen gas, while a second pulse heated the gas to plasma. The combination prepared the way for a short, 10-trillion-watt (10 TW) driver pulse from a separate, chirped-pulse laser. In this way LOASIS produced a high-quality, 80-million-electron-volt (80 MeV) electron beam within a distance of a few millimeters.
If in 2004 the entire world was consuming a dozen terawatts of power every second, how could a single laser in a small laboratory put out that much power or more? By packing an otherwise modest amount of energy into a much shorter slice of time: a mere half a joule of energy concentrated in 40 or 50 femtoseconds - the time it takes light to travel a third the width of a human hair - delivers 10 to 15 TW of peak power. The LOASIS researchers built the drive laser themselves and named it Godzilla. (They also built the drill and heater laser, named Chihuahua.)
By 2006 LOASIS was a full-fledged program in AFRD. The plasma channel had evolved to a physical channel in a block of sapphire, a technology pioneered by Simon Hooker and his group at Oxford University; an electrical discharge heated the gas inside to plasma. Using another “handmade” laser, this one with 40 TW peak power and named T-REX, the LOASIS crew achieved a high-quality beam of electrons with an energy of a billion electron volts (1 GeV) in a distance of just 3.3 centimeters, a little over an inch. A typical linear accelerator would require over two-thirds the length of a football field - 2,000 times as far - to reach the same energy.
Brighter beams and higher energies
Chirped-pulse amplification has so revolutionized the optics industry that today 10-TW lasers can be bought commercially for $1 million; 100-TW lasers are only about twice as much, an astonishing bargain by the standards of just a few years ago. But LOASIS is aiming higher.
Working with the private sector on a petawatt (quadrillion watt) laser system, one capable of delivering 40 joules of energy once every second in 40-femtosecond pulses, LOASIS is building the Berkeley Lab Laser Accelerator (BELLA) to accelerate a high-quality beam of electrons to 10 billion electron volts (10 GeV) in less than a meter. The U.S. Department of Energy has signaled its commitment to this quest for the accelerator of the future by granting BELLA a spate of simultaneous approvals.
Although it sounds like alphabet soup, DOE’s Critical Decision scheme is straightforward: once it has been agreed that there’s actually a need for a new thing, which DOE calls Critical Decision 0, CD-0, next comes the conceptual stage, CD-1, when a specific approach and cost range are determined. Then CD-2 looks at technical specifications to judge whether the project can be completed on time and on budget, CD-3 approves the start of construction, and finally CD-4 flips the “on” switch.
A high-priority project may move ahead on several fronts at once, developing detailed plans for some aspects while alternatives are still being evaluated for others. Meanwhile, construction can start on some essential components. That’s the case with BELLA, which received simultaneous CD-1, CD-2A, and CD-3A approvals in September of 2009.
“CD-2A/3A gives the approval to start the long process of procuring and constructing the laser systems, whose cost is well established,” says Sergio Zimmermann of the Engineering Division, BELLA’s project manager, “while CD-1 gives the BELLA team the go-ahead to design the remaining systems beyond the conceptual stage.”
BELLA’s chief aim is to accelerate electrons to 10 GeV in a one-meter module, but at the same time LOASIS is continuing research and development on equally important questions with its current lasers. There’s a limit to the distance over which a laser beam can retain critical focus, so a laser plasma accelerator, like a powerful conventional accelerator, will need many accelerating modules, each boosting the energy of the beam as it passes from one to the next.
LOASIS scientists are using the existing 3.3-centimeter modules to investigate injection and staging schemes for carrying well-formed bunches of electrons from one module to the next, while introducing the laser pulses needed to further focus and accelerate the bunches in close synchronization.
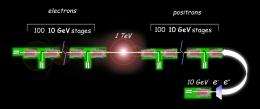
Sometimes called “tabletop” accelerators, a moniker that conveniently ignores the extensive laser systems needed to drive the electrons inside the accelerating module, laser plasma accelerators will nevertheless revolutionize high-energy physics. Once the challenges of staging and injection are mastered, hundreds of accelerating modules can be linked to produce electron colliders reaching center of mass energies in the trillions of electron volts (TeVs) - not in the scores of kilometers needed for conventional machines but in half a kilometer or less.
Other challenges remain. At one pulse per second, BELLA’s petawatt laser will have a high repetition rate for a big laser, but that’s a million times slower than what the NGLS electron injector aims for. Nevertheless, BELLA will be capable of unique science in its own right. The BELLA laser accelerator may form the core of a user facility unlike any in the world for research in physics, chemistry, biology, and materials.
For example, by colliding a 10-GeV electron beam with a separate petawatt laser pulse, extraordinarily high electric fields could be created in which accelerated electrons could gain energy equivalent to their own rest mass - enough to create electron-positron pairs out of the vacuum. It’s a phenomenon known as “snapping” or “boiling” the vacuum, a regime more familiar to astrophysicists than laser scientists.
Intense electron beams up to 10 GeV, as short as a femtosecond in duration, plus petawatt-peak-power laser beams; it’s a combination that will drive accelerator science in whole new directions - inside the laboratory.
Return to Accelerators and Light Sources of Tomorrow, Part 1: From Linacs to Lasers
More information:
-- Learn more about LOASIS
-- The LOASIS program home page
-- More about the science behind BELLA
This feature story is republished with permission.
Provided by Lawrence Berkeley National Laboratory