How zinnias shaped a budding biologist, and other fun facts about plants
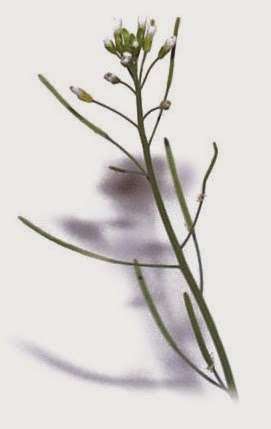
"I've always been really fascinated by plants, even from a young age," says Emory biologist Roger Deal. "Their lives are so interesting, even though they are stuck where they are born."
Deal's roots are in Columbia, South Carolina, where his father was a physician and his mother loved to garden.
"My first plants were zinnias," he recalls. "I was about 10 and my mom and I went to the garden store where I picked out a packet of seeds. You have these little dry things that look like pieces of dust. All you have to do is put them in the ground and get them wet, and then you have a whole organism. I thought, 'Wow, what an amazing life cycle! How does it work?'"
Plants go back millions of years, when the Earth's atmosphere contained very little oxygen. "Where did all that extra oxygen come from? It's a byproduct of plants," Deal says. "All the energy that we need to live also comes from plants. And they're beautiful – they're an important part of our aesthetic. We are basically tied into plants in myriad and intricate ways."
As an undergraduate at the University of South Carolina, Deal worked in a lab that studied phytoremediation, or the process of using plants to clean up pollution. "A lot of plants are tolerant of heavy metals," he explains. "I worked on a project that was exploring how to use Spartina, the grass you see growing along salt marshes on the coast, to suck mercury pollution up out of the soil."
Deal became interested in how genes are controlled in plant development during his graduate school years at the University of Georgia.
In his lab at Emory, he's continuing this focus on how plants build and adapt their bodies. By digging deep into the developmental biology and genetics of plant systems, he hopes to unearth secrets that could benefit both agriculture and human health.
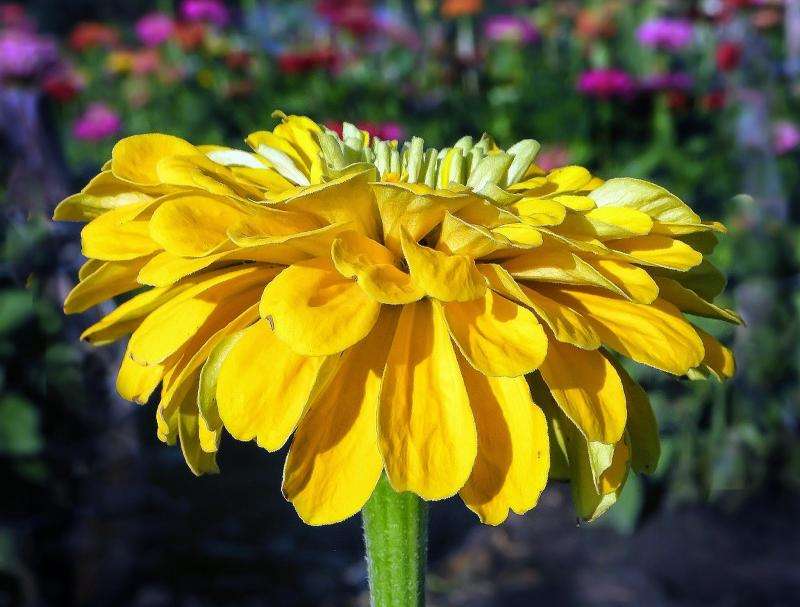
"A big question in studying the genome of any organism is figuring out where all the genes are, and how you put all the parts together to build an organism," he explains. "Humans have a parts list of about 30,000 different genes, for example, but only 2 percent of our genome is genes. The rest was once considered junk DNA, but we now know that it's not junk."
DNA is not just floating around by itself in the nucleus of a cell. It's wrapped up in little globules of proteins called histones. By wrapping tightly around histones, a six-meter long strand of DNA can cram into a cell nucleus.
"This complex of histones and DNA is called chromatin," Deal says. "The chromatin is used to turn genes on or off, which determines the function of a cell. So chromatin is part of the system that differentiates the cells when an embryo is growing. Chromatin is also involved in establishing and maintaining cell proliferation. If cell proliferation gets turned on inappropriately, the result can become a tumor."
One of the model organisms Deal's lab uses is Arabidopsis thaliana, a small flowering plant that is a member of the mustard family. "It's just a lowly little weed that you've probably stepped on and not noticed," Deal says of Arabidopsis. "It grows all over the northern hemisphere."
The Arabidopsis genome is about one-twentieth the size of the human genome. "It's sort of streamlined," Deal says. "It has about the same number of genes as we do, but it has way less 'intergenic' DNA. So in terms of finding the regulatory parts, we have a lot less stuff to look at. When it comes to lab research, Arabidopsis is like the fruit fly of the plant world."
Arabidopsis plants begin their life as the fusion of a sperm and an egg: A single cell, which develops into an embryo inside of a seed. "This little embryo can sit there for decades," Deal says. "But once it gets wet, the whole thing kicks into action. Suddenly, it starts growing, pops a root, develops leaves."
At some point, the plant switches from vegetative growth to reproductive growth. "Something in the environment, the length of the day, the quality of light, tells these plants it's time to stop making leaves and start making flowers," Deal says. "A really important question is how this switch operates at the molecular level."
A key part of the puzzle appears to be a histone protein called H2AZ, which is an important component of chromatin in plants, animals and fungi.
"The H2AZ gene is essential for life in animals," Deal says. "If an embryo doesn't have it, the embryo stops developing and dies. But an overproduction of an H2AZ molecule appears to drive the genesis of several types of cancer, including breast and prostate cancer."
Plants, unlike laboratory rats, can survive without the H2AZ gene. "If you knock out the H2AZ gene in Arabidopsis plants they don't die, it just messes them up," Deal says. "The lack of H2AZ affects the leaf size, flowering time and their susceptibility to pathogens and other stresses."
That makes Arabidopsis an easily accessible model to study this particular gene and its on-and-off switch. "We can mess with Arabidopsis in pretty extreme ways, removing this critical regulator of a process, and then study what happens at the molecular level," Deal says. "We're hoping that our research will help us understand something about the biology of cancer and the biology of animal and plant development all in one fell swoop."
Provided by Emory University