March 3, 2015 report
The origins of polarized nervous systems
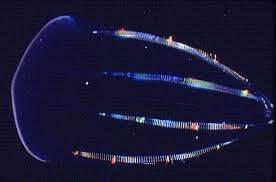
(Phys.org)—There is no mistaking the first action potential you ever fired. It was the one that blocked all the other sperm from stealing your egg. After that, your spikes only got more interesting. Waves of calcium flooding the jointly-forged cell stiffened its glycoprotein-enhanced walls against all other suitors and kicked off the developmental program ultimately responsible for constructing your brain. Unlike the nervous systems of the lower forms of life, our neurons have a clearly polarized form—a single output axon projecting far to parts unknown is charged by input dendrites feeding on the local metabolic soup de jour. The origins of this polarity in neurons, and therefore in nervous systems in general, are written in the primitive body plans of the mostly gelatinous organisms still hailing intact across deep time.
How a blunt multipurpose neuronal toolkit that originally evolved to nourish was morphed into the precise cellular utensils we now use to mince the world is the subject of a new special issue in the Journal of Experimental Biology (JEB). The story of the acquisition of polarity, or rather the loss of universality, in the flow of resource through crude nerve nets is the story of our brains. Last year,Tim Jegla from Penn State published work showing that the human Erg potassium channels that are tuned to repolarize the long action potentials underlying the strong muscular contractions of our hearts have their origins in the earliest nervous systems ever evolved.
Since then he has been piecing our brains together by tracing the evolution of related channels like the EAG potassium channels, and the so-called Shaker potassium channels in various primitive organisms. The creatures that have been the most informative mostly fall into taxonomic groups of typically radially-symmetric animals named with a strange variation on the letter C. The 'cnidarians' are animals like hydras and the true jellyfish, while the 'ctenophores' are the comb jellies that swim with cilia.
In an article on polarity written together with Melissa Rolls, Tim explores how the positioning of different kinds of channels by the cytoskeleton (at places like the axon initial segment, nodes, and dendrites) is crucial for establishing directional signalling in neurons. So I asked him point blank if he could nail down when polarity first evolved. He said it was likely in an ancestor of the parahoxozons, a group defined by their possession of at least one of the Hox/ParaHox genes associated with the the specification of the body axis. With genetic experiments now in progress in his lab Jegla is looking for indications that polar neurons exist, contrary to the current literature, even in the lowly sea anemone. As cnidarians, anemone are privy to the benefits of parahoxozoans, something the ctenophores cannot claim.
In looking for larger developmental trends in which to anchor the idea of increased polarity, or loss of flexibility in neurons, ctenophores may have other secrets to tell. One author writing in the special issue of JEB suggests that recent whole genome data puts ctenophores as a sister group to all other animals, placing them at the earliest branching lineage—a move which would make them a more basal metazoan than even SpongeBob himself. Two factors which complicate such analysis are convergent evolution and the loss of genes and function. We might imagine that the possibility of having at least two independent origins for neural systems exists regardless of which lineage was prior.
It has been known since the work of Chun in 1880 that when ctenophore blastomeres are separated at the two-cell stage each half-embryo develops exactly half of adult structures. It seems that this high degree of determinism at the organism level, which fades in the progression of species, contrasts with the aquisition of specification at the cell level. Among the important proteins known to exist in these primitive organisms are various kinds of G-protein coupled receptors and gap junctions. Originally it looks like these proteins played important roles in cell adhesion and communication, and therefore in early development and specification of the body plan.
Enzymes to synthesize and transport neurotransmitters were also present early on. A trend in moving to more advanced body plans, and neurons, is the restriction of the expression of these transmitter systems to specific cells. On top of this there is an inexorable refinement of multipurpose symmetric synapses into asymmetric synaptic diodes, with concommitant exclusivity of transmitter profile in both dense core and clear vessicles. In creature like planarians, worms, or flies, the percentage of neurons we might call 'polar' becomes an increasingly important thing for us to take account of. In C. elegans for example, many neurons, with the exception of the elaborate and highly branched sensory neurons that span the whole body, are fairly simple with just a few processess containing synapses that can be both pre and post synaptic.
The neurons of many insects, like drosophila, are conspicuous for their dense regions of idiosyncratic branching where the terms dendrite or axon would seem to have little meaning. While the neurons of higher and larger vertebrates are expected to in a sense 'feel' every spike they might pull off throughout the whole neuron, it paradoxically seems that tiny invertebrate neurons none-the-less are more likely to contain isolated domains of protected metabolic and electrical activity. The details of all this are to be found at the molecular level, which at this time in the history of neurobiology means the the directions of the 'plus' and 'minus' ends of various cytoskeletal proteins, and the preferences of the motors that ride them in either direction.
Like vertebrates, drosophila have axons that exclusively use plus-end-out microtubules. However, although their dendrites similarly are distinguished by the presence minus-end-out microtubules, one surprise was that almost all of their dendrites were this way. The kicker is that they start out with a even mix like vertebrates do, but over time somehow weed them out. An important element in any potential theory of neurons would be the role of the cell body (the nucleus, centriole and primary cilium) in the ongoing specification of the larger tree of axons and dendrites. A cell body that stands largely aloof from them, whether transiently or permanently, would appear to lose some of the authority it might have if interposed instead between them.
The unique geometry of the pseudounipolar neurons of our dorsal root ganglion has been known for a while, but only more recently has the frequent presence of axons sprouting from dendrites in our hippocampus and cortex been appreciated. In seeking explanations for the structure of such neurons the influences of mitochondria in various states of performance and lifecycle should not be underestimated. In fact, mitochondria figure importantly in the entire evolutionary curiosity we have tried to lay out here. The same article above which stirred up question surrounding the primitive phylogeny of sponges also explored newly identified trends that emerge in looking at the sizes and contents of the genomes of mitochondria across metazoans—but that is probably a topic sufficient for another post.
More information: Journal of Experimental Biology, jeb.biologists.org/content/current
Journal information: Journal of Experimental Biology
© 2015 Phys.org