Superconductivity without cooling
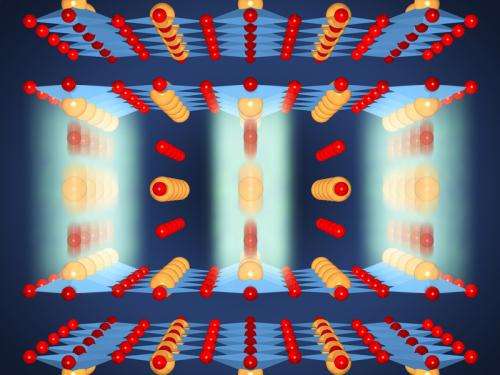
Superconductivity is a remarkable phenomenon: superconductors can transport electric current without any resistance and thus without any losses whatsoever. It is already in use in some niche areas, for example as magnets for nuclear spin tomography or particle accelerators. However, the materials must be cooled to very low temperatures for this purpose. But during the past year, an experiment has provided some surprises.
With the aid of short infrared laser pulses, researchers have succeeded for the first time in making a ceramic superconducting at room temperature – albeit for only a few millionths of a microsecond. An international team, in which physicists from the Max Planck Institute for the Structure and Dynamics of Matter in Hamburg have made crucial contributions, has now been able to present a possible explanation of the effect in the journal Nature: The scientists believe that laser pulses cause individual atoms in the crystal lattice to shift briefly and thus enhance the superconductivity. The findings could assist in the development of materials which become superconducting at significantly higher temperatures and would thus be of interest for new applications.
In the beginning, superconductivity was known only in a few metals at temperatures just above absolute zero at minus 273 degrees Celsius. Then, in the 1980s, physicists discovered a new class, based on ceramic materials. These already conduct electricity at temperatures of around minus 200 degrees Celsius without losses, and were therefore called high-temperature superconductors. One of these ceramics is the compound yttrium barium copper oxide (YBCO). It is one of the most promising materials for technical applications such as superconducting cables, motors and generators.
The YBCO crystal has a special structure: thin double layers of copper oxide alternate with thicker intermediate layers which contain barium as well as copper and oxygen. The superconductivity has its origins in the thin double layers of copper dioxide. This is where electrons can join up to form so-called Cooper pairs. These pairs can "tunnel" between the different layers, meaning they can pass through these layers like ghosts can pass through walls, figuratively speaking – a typical quantum effect. The crystal only becomes superconducting below a "critical temperature", however, as only then do the Cooper pairs tunnel not only within the double layers, but also "spirit" through the thicker layers to the next double layer. Above the critical temperature, this coupling between the double layers is missing, and the material becomes a poorly conducting metal.
The result helps material scientists to develop new superconductors
In 2013, an international team working with Max Planck researcher Andrea Cavalleri discovered that when YBCO is irradiated with infrared laser pulses it briefly becomes superconducting at room temperature. The laser light had apparently modified the coupling between the double layers in the crystal. The precise mechanism remained unclear, however – until the physicists were able to solve the mystery with an experiment at the LCLS in the US, the world's most powerful X-ray laser. "We started by again sending an infrared pulse into the crystal, and this excited certain atoms to oscillate," explains Max Planck physicist Roman Mankowsky, lead author of the current Nature study. "A short time later, we followed it with a short X-ray pulse in order to measure the precise crystal structure of the excited crystal."
The result: The infrared pulse had not only excited the atoms to oscillate, but had also shifted their position in the crystal as well. This briefly made the copper dioxide double layers thicker - by two picometres, or one hundredth of an atomic diameter - and the layer between them became thinner by the same amount. This in turn increased the quantum coupling between the double layers to such an extent that the crystal became superconducting at room temperature for a few picoseconds.
On the one hand, the new result helps to refine the still incomplete theory of high-temperature superconductors. "On the other, it could assist materials scientists to develop new superconductors with higher critical temperatures," says Mankowsky. "And ultimately to reach the dream of a superconductor that operates at room temperature and needs no cooling at all." Until now, superconducting magnets, motors and cables must be cooled to temperatures far below zero with liquid nitrogen or helium. If this complex cooling were no longer necessary, it would mean a breakthrough for this technology.
More information: "Nonlinear lattice dynamics as a basis for enhanced superconductivity in YBa2Cu3O6.5." Nature 516 71, DOI: 10.1038/nature13875
Journal information: Nature
Provided by Max Planck Society