This article has been reviewed according to Science X's editorial process and policies. Editors have highlighted the following attributes while ensuring the content's credibility:
fact-checked
peer-reviewed publication
trusted source
proofread
Researchers achieve first condensation of non-ground state cesium atoms
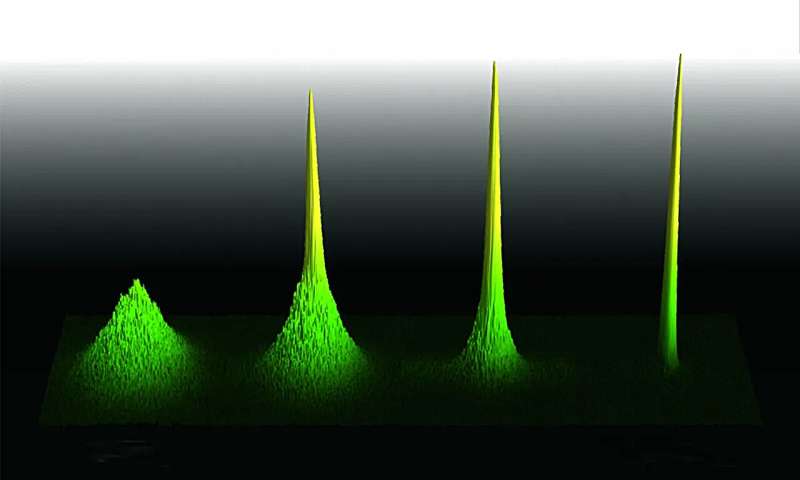
In a pioneering effort, researchers from the University of Innsbruck in collaboration with the University of Durham have for the first time achieved Bose-Einstein condensation of non-ground state cesium atoms. Published in Nature Communications, this research paves the way for new experiments with ultracold atomic gases and the study of many-body quantum physics.
The world of atoms, typically characterized by random chaos and heat, undergoes a remarkable transformation when atoms are drastically cooled. At temperatures just above absolute zero, atoms enter a unique quantum state known as a Bose-Einstein condensate (BEC), where they behave as a single, coherent entity. The first successful realization of a BEC was in 1995, 70 years after the theoretical prediction by Albert Einstein and Satyendra Nath Bose.
Since then, researchers have been delving into the peculiar properties of these ultracold gases to unravel mysteries of quantum mechanics. Moreover, ultracold atomic gases, known for their high degree of controllability, have served as invaluable testbeds for few- and many-body quantum physics.
Cesium, in particular, has been instrumental in this regard due to its rich landscape of Feshbach resonances, enabling precise tuning of interactions. Traditionally, cesium has been condensed in its absolute ground state. Now, researchers from the University of Innsbruck, in collaboration with a theory team from the University of Durham, have for the first time achieved the condensation of cesium atoms in the Zeeman-excited mF=2 state, a non-ground state configuration.
"The attainment of Bose-Einstein condensation hinges upon maintaining a favorable ratio of good to bad collisions. Elastic collisions play a crucial role in driving the evaporation and thermalization process, while two-body inelastic collisions and three-body recombination can diminish the cooling efficiency, possibly to the point that BEC cannot be reached," explains Milena Horvath, the first author of the study.
The team identified two distinct magnetic field regions where condensation is possible, with negligible two-body losses and sufficiently suppressed three-body losses. "Condensing cesium atoms in this non-ground state configuration has also revealed some interesting and unexpected three-body loss mechanisms," says Horvath.
"The discovery of unexpected three-body loss mechanisms highlights the intricacies of ultracold atom systems and underscores the importance of detailed experimentation," adds lead scientist Hanns-Christoph Nägerl.
This latest achievement builds upon two decades of progress since cesium was first condensed in Innsbruck in 2003, showcasing the ongoing advancements in the field. "This accomplishment adds to the rich history of quantum research in Innsbruck," says Hanns-Christoph Nägerl.
"As we continue our journey, we look forward to deepen our understanding of many-body quantum physics such as impurity and polaron physics, as well as topological phase transitions and quantum-gas mixtures."
More information: Milena Horvath et al, Bose-Einstein condensation of non-ground-state caesium atoms, Nature Communications (2024). DOI: 10.1038/s41467-024-47760-0
Journal information: Nature Communications
Provided by University of Innsbruck