September 25, 2023 feature
This article has been reviewed according to Science X's editorial process and policies. Editors have highlighted the following attributes while ensuring the content's credibility:
fact-checked
peer-reviewed publication
trusted source
proofread
Scientists observe Hubbard exciton in strongly correlated insulators
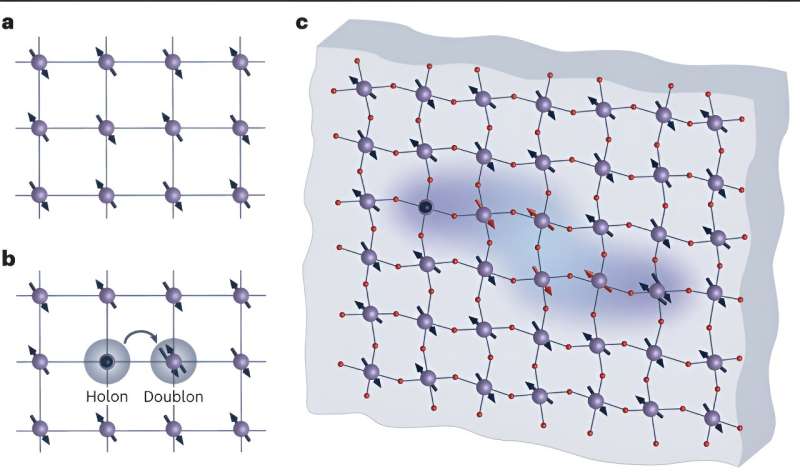
A team of scientists, led by Dr. David Hsieh from the California Institute of Technology, have observed evidence of stable Hubbard excitons in a photo-doped antiferromagnetic Mott insulator. The findings of their study are published in Nature Physics.
In the world of condensed matter physics lies an exciting phenomenon that has long been a subject of interest—excitons.
Typically found in semiconductor materials, excitons are composite particles (or quasiparticles) that emerge when an electron and a hole—the absence of an electron—interact through electrostatic forces.
Excitons, akin to the proton and electron in a hydrogen atom, exist fleetingly and have been well-studied. However, their behavior changes when we enter the realm of Mott insulators, materials that defy the conventional rules governing electron behavior in ordinary semiconductors.
It has long been theorized that the Hubbard exciton, a novel quasiparticle, may emerge in Mott insulators. But whether or not they can actually exist as stable quasiparticles in real materials has remained an open question. Now, scientists have experimental data suggesting that the answer is yes.
Explaining his team's motivation to study these systems, Dr. Hsieh told Phys.org, "Our research group is generally interested in materials that feature strongly interacting electrons, particularly in far-from-equilibrium settings."
Mott insulators, charges and antiferromagnetism
The intrinsic properties of Mott insulators are responsible for the Hubbard exciton differing from conventional excitons in semiconductors.
Unlike semiconductors where electrons are delocalized, Mott insulators force electrons into specific lattice sites due to strong Coulombic interactions. This localization creates a distinct bandgap, contrasting with traditional semiconductors where electrons move more freely.
Dr. Hsieh explained, "In this state, it is favorable for the electron spins to arrange themselves in an alternating orientation from one site to the next, resulting in a pattern dubbed antiferromagnetic order.
"This antiferromagnetic environment creates the possibility of realizing the Hubbard exciton."
Antiferromagnetic interactions are essentially magnetic interactions where neighboring electron spins align in opposite directions, leading to a unique magnetic order within the material.
Understanding and detecting Hubbard excitons is more challenging than regular excitons in semiconductors. This is due to the complex interplay of electronic interactions, the antiferromagnetic order of the material, and the short-lived nature of these excitons.
Dr. Hsieh elaborated, "Experimentally, they are difficult to detect using traditional spectroscopic techniques that have been used for regular semiconductor excitons. This is why we employed a stroboscopic technique to capture the exciton formation in real-time."
Searching for fingerprints
Led by former graduate student Omar Mehio, the team's experimental setup hinged on the choice of the Mott insulating material and the spectroscopy technique.
Choosing the correct material was paramount, and the team chose Sr2IrO4, an antiferromagnetic Mott insulator known for its intricate electronic interactions.
For the spectroscopic measurements, the team chose terahertz radiation, which are low-frequency waves ideal for probing the internal structure of excitons.
The team began by using an infrared laser pulse to excite the charge carriers across the bandgap. The terahertz spectrometer then allowed them to capture the transient response of Sr2IrO4 in real time to detect the spectra, or distinctive fingerprint, of the Hubbard exciton.
Initially, this material, typically behaving as an insulator, transitioned into a conductor-like state, as expected, given the generation of free electrons and holes.
But rather than simply returning to the original insulating state, the material rapidly transitioned to a different kind of insulating state, one with a spectrum more akin to that of an atom. Unlike the spectra of hydrogen, however, the energy level spacing is only a few millielectronvolts, as expected for a Hubbard excitonic fluid.
These findings, supported by advanced computer simulations performed by Dr. Zala Lenarcic at the Jozef Stefan Institute, provided compelling evidence of the existence of a Hubbard excitonic fluid within Sr2IrO4.
However, the experiment was not without its challenges. Dr. Hsieh highlighted some of these, saying, "We faced several hurdles, including growing large, high-quality crystals to accommodate the terahertz beam (courtesy of Dr. Stephen Wilson at U.C. Santa Barbara) and adapting to reflection geometry to overcome the effects of material absorption.
"Additionally, the signal from the Hubbard exciton is rather weak. So, we had to suppress sources of noise in our measurements, like fluctuating lab temperatures."
What's next?
The characterization of Hubbard excitons in strongly correlated insulators, as demonstrated by Dr. Hsieh and his team, opens up a realm of exciting possibilities for both fundamental understanding and practical applications.
"It is difficult to predict potential technological applications," said Dr. Hsieh, "One might imagine new functionalities where the excitonic properties can be tuned through magnetic degrees of freedom or where excitonic properties (for optical applications) and magnetic properties (for information storage applications) can both be exploited in a single material."
Future research can delve into fundamental questions about Hubbard excitons, such as their binding mechanisms and interactions with different magnetic states.
Dr. Hsieh and his team aren't done with Hubbard excitons yet. Sharing his plans for the next steps, he said, "There are many remaining questions about Hubbard excitons of fundamental importance that we plan to pursue. Questions at the top of our minds include the following. Can we prove that the Hubbard exciton is indeed predominantly bound by antiferromagnetic interactions? How do the Hubbard exciton properties change with the texture of the underlying magnetic state? What is the nature of the interactions between Hubbard excitons, and what new collective excitonic phases might they generate?"
The journey to unlock the full potential of Hubbard excitons has just begun, and it may hold the promise of reshaping our understanding of quantum phenomena.
Edoardo Baldini has published a News & Views piece in the same journal issue on the work by Hsieh's team.
More information: Omar Mehio et al, A Hubbard exciton fluid in a photo-doped antiferromagnetic Mott insulator, Nature Physics (2023). DOI: 10.1038/s41567-023-02204-2
Edoardo Baldini, Charges tied with magnetic strings, Nature Physics (2023). DOI: 10.1038/s41567-023-02187-0
Journal information: Nature Physics
© 2023 Science X Network