December 19, 2018 feature
Self-healing electroluminescent (EL) devices
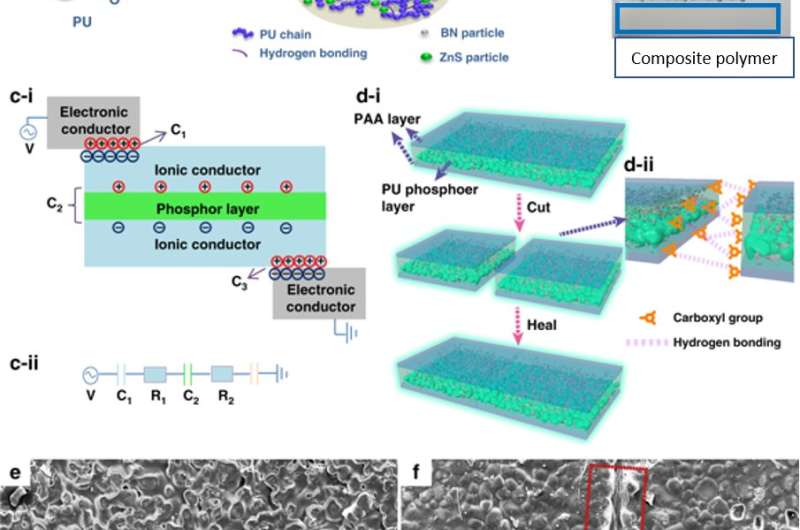
In a recent study, materials scientists Guojin Liang and his coworkers at the Department of Materials Science and Engineering, City University of Hong Kong, have developed a self-healing, electroluminescent (EL) device that can repair or heal itself after damage. Inspired by the self-healing capacity of biological systems, the novel process paves the way for a range of new electronic applications. While EL devices are typically used in digital displays, backlights for control panels and wearable electronics, they are prone to damage. The fragility can restrict device working life, sustainability and reliability for long-term electronic applications.
The first self-healing EL device developed in the study was constructed by using a modified self-annealing polyacrylic acid hydrogel for electrodes, coupled to self-healing polyurethane as a phosphor host for electric insulation. The scientists demonstrated that the physiochemical properties of device restoration could be maintained even after experiencing catastrophic damage. Such EL systems will have new and exciting next-generation applications as healing hydrogels and dielectric polymers in wearable devices. The work is now published on Light: Science & Applications.
The luminescent performance of self-healing EL devices were recovered with high healing efficacy in the study. The concept could be transferred to inter-device healing to realize a conceptual LEGO-like assembly process in light-emitting devices. Through the design and engineering process of self-healing EL devices, the scientists aimed to revive their performance while expanding their lifetime, even after the material was cut in half. Liang et al. expect such self-healing EL devices developed using ionically conductive healing hydrogels and dielectric polymers to serve as a model system for electroluminescent applications.
Electroluminescent (EL) devices have applications in versatile systems and disciplines including soft robotics, as artificial skin actuators; in flexible electronics, wearable electronics, digital displays and as sensors. Advances in materials science have led to exquisitely designed and developed multi-functional EL devices using transparent, conductive materials that integrate biologically inspired soft robotics and optimized device configurations. Examples include:
- Stretchable robots with cephalopod-inspired camouflage.
- Silver nanowires for transparent electrodes in self-deformable EL actuators.
- Extremely stretchable EL devices engineered with ionically conductive hydrogels, and wearable fabrics and sensors for optical communication.
Although mechanical engineering processes have developed robust devices with robust materials, for device configurations that minimize strain, if strain exceeds the withstanding limit, degradation of device performance cannot be avoided. The maintenance and replacement of such faulty components in integrated, multi-functional electronic systems is either intractable or costly, and remains a primary concern. Developing an effective strategy can significantly extend the lifetime of EL devices.
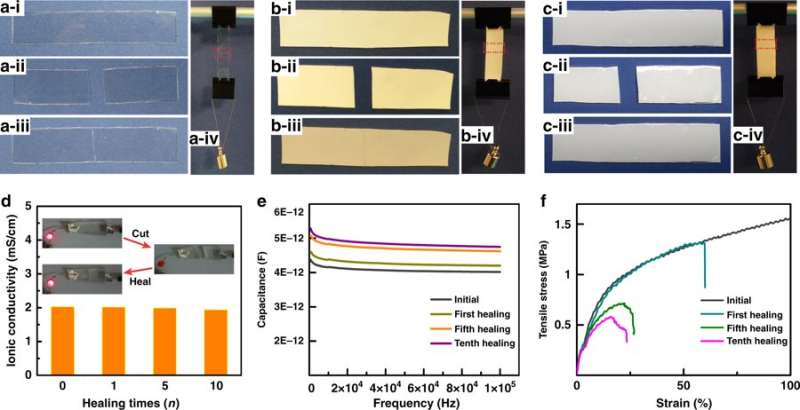
In the study, self-healing materials were designed and developed with intrinsic or extrinsic healing features, similar to previous experiments. The present features allow autonomous damage repair, even under external stimuli such as pH, light, electric or magnetic fields after extensive damage. Healing properties exist at the level of the material, but improvements to enhance device lifetime and reliability are ongoing. A majority of previous self-healing performances were also only applicable at the level of a single constituent layer in a multilayered EL device, where the remaining layers were manually repaired via small patches.
To address existing limitations, Liang et al. adopted modified self-healing polyacrylic acid (PAA) hydrogels containing sodium chloride (NaCl) as an ionic electrode. Then they included self-healing polyurethane (PU)-containing zinc sulfide (ZnS) particles as a phosphor composite layer to demonstrate the first innately optimized self-healing EL device in the study. Alongside fracture damage healing, inter-device healing was also restored for the first time to allow LEGO-like assembly at the level of the EL device. The study simplified complex and costly processes of repair and replacement in integrated electronic systems.
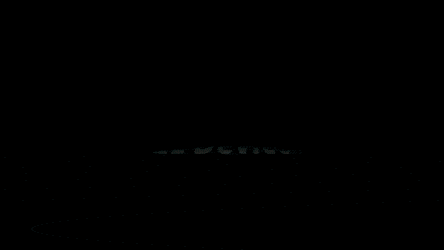
The polymer-based hydrogels (PAA layer and PU composite layer) were developed at the molecular level to obtain the desired physicochemical character. A hydrogel was engineered to simultaneously realize the desired traits of visible-light transparency, ionic conductivity and self-healing. The PAA hydrogel contained uniformly distributed NaCl (4M) to achieve 96.5 percent average transmittance for transparency in the range of visible light and a high ionic conductivity of 2.1 S/m. The carboxyl groups on the PAA backbone transferred self-healing capability to the hydrogel via hydrogen bonding.
The electrically insulating, light-emitting and self-healing phosphor composite layer contained PU modified with carboxyl groups to bind phosphor particles (ZnS). The scientists used boron-nitride (BN) nanosheets to increase dielectric permittivity and enhance luminescence of the composite layer. The final EL device comprised a three-layered configuration. The materials were assessed using scanning electron microscopy (SEM) after fabrication and after the damage/healing response. The physicochemical character of the multi-layered material was restored after damage.
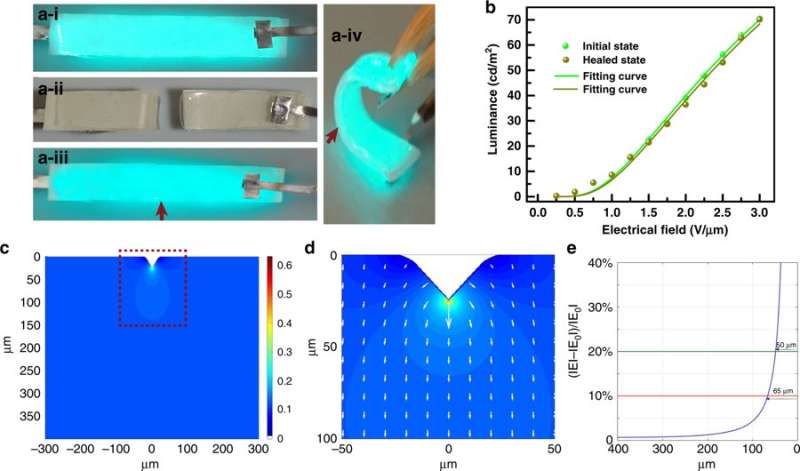
The EL device contained the composite phosphor layer (composed of PU/ZnS/BN) sandwiched by two symmetric (PAA-NaCl based) transparent ionic conductors. The morphology of each layer/component was characterized using field-emission SEM and optical images. Restoration of mechanical strength after healing each layer was evaluated by hanging a weight. Ionic conductivities that were measured with electrochemical impedance spectroscopy (EIS) showed full restoration compared to the initial value, even after 10 cutting-healing cycles.
As proof of working principle, the scientists also observed the revival of a light emitting diode (LED) circuit after cut-healing cycles of the conducting material. Capacitance of the dielectric layer remained almost constant after different healing cycles, indicating that the light emission intensity and distributed voltage remained almost constant after healing. Mechanical properties of the healed EL device indicated restoration of both tensile strength and Young's modulus at the device breaking point, even after 10 cutting-healing cycles.
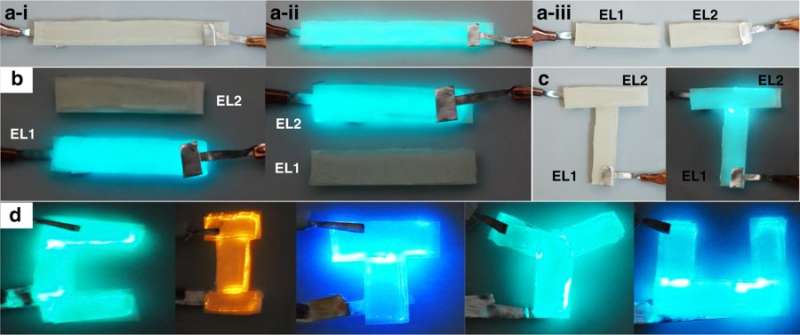
The scientists also demonstrated the initial working state, cutting state and healed state (work after healing) guided by the rational design for both materials and the device. Successful revival of the light-emitting performance of the EL device indicated restoration of physicochemical and mechanical properties in each functional layer. The experimental data fit well with the equation derived in the study for both initial working state and the healed state.
Based on the outstanding healing capacity of the EL devices, the scientists assembled a few EL units into one integrated EL system to build a LEGO assembly of light-emitting devices. To accomplish this in the lab, the EL device was cut in half to create EL1 and EL2, and both units worked without any visible degradation in luminescence. During the LEGO assembly process, the scientists implemented two EL units to form a T-shaped light-emitting letter, with visible functionality on healing. Additionally, the scientists assembled the materials to form words with colorful light emissions using ZnS phosphor particles doped with different elements.
The study was a first to report the arbitrary LEGO-like assembly of EL devices, where all functional layers could be healed. The novel design can be applied to develop ionically conductive, healing hydrogels as transparent electrodes and healing dielectric polymers with insulating phosphor layers. Such materials will have advanced applications to build the next generation of wearable, deformable and self-healing electronics.
More information: Guojin Liang et al. Self-healable electroluminescent devices, Light: Science & Applications (2018). DOI: 10.1038/s41377-018-0096-8
Yu Shrike Zhang et al. Advances in engineering hydrogels, Science (2017). DOI: 10.1126/science.aaf3627
Cheng-Hui Li et al. A highly stretchable autonomous self-healing elastomer, Nature Chemistry (2016). DOI: 10.1038/nchem.2492
C. Keplinger et al. Stretchable, Transparent, Ionic Conductors, Science (2013). DOI: 10.1126/science.1240228
Journal information: Light: Science & Applications , Nature Chemistry , Science
© 2018 Science X Network