Controlling interactions between distant qubits
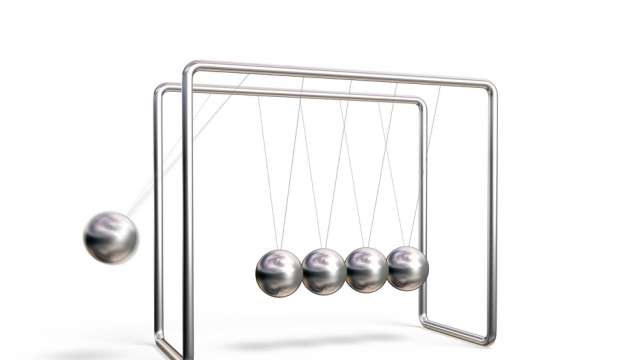
A big part of the burgeoning science of quantum computation is reliably storing and processing information in the form of quantum bits, or qubits. One of the obstacles to this goal is the difficulty of preserving the fragile quantum condition of qubits against unwanted outside influence even as the qubits interact among themselves in a programmatic way.
Spin qubits are one of the most promising candidates for the purpose. Besides being charged, electrons possess spin, a kind of magnetic axis that can only assume specific quantized values. An atom with a single unpaired electron can serve as a qubit if that electron can be tickled into residing in both of two allowed quantum states (usually called spin up and down) at the same time. Likewise, a carefully contrived small puddle of electrons known as a quantum dot can also serve as a qubit. The dot's spin consists of the aggregate spin of the small number of electrons (two, three, four, etc.) residing in the dot. In this way the dot acts as a sort of artificial atom.
JQI scientists, publishing in Physical Review Letters, show how both of these qubit types—-atomic spins and quantum dot spins—-can be combined into a workable quantum system. According to Jacob Taylor, the leader of the JQI work, their suggestion for a new qubit interaction protocol uses the dot's spin to turn on what is essentially a chemical reaction between two atomic spins which sit as much as 50 or 100 nm apart. In the proposed scheme, the atoms designated for qubit duty are phosphorus impurities sitting in a silicon crystal.
Qubit chemistry
Why chemistry? Well, according to Taylor a large part of chemistry is the reaction of two chemical species via their outer electrons by forming a temporary or stable chemical bond governed by the Pauli exclusion principle. This principle says that two identical electrons cannot occupy the same position. This aversion—-a purely quantum property of nature—-acts in addition to the ordinary electrostatic repulsion between like-charged particles.
The direct electrical connection between otherwise identical electrons, usually called the exchange interaction, depends on the relative spin orientation of the participating electrons. The Pauli exclusion effect will lead to greater repulsion for electrons with the same spin. The repulsion, the inability of electrons to interpenetrate, accounts for much of the "stiffness" of matter. For instance, you can't push your hand through a solid desk because, at the microscopic level, electrons are repelling other electrons.
"Much of chemistry operates via the exchange interaction," says Taylor. "But this interaction is too tenuous to operate effectively between the distant atomic spins. The compromise is to use a largish quantum dot—-acting like at atom but having a size corresponding to the distance between the impurity atoms—-to link up the atoms."
The central metaphor for this scheme, Taylor says, is Newton's cradle, a device consisting of several identical balls suspended from thin wires. Lift one ball up, let it go, and it will collide with the neighboring ball. The kinetic energy and momentum of the first ball, passed on to the second, will be passed smoothly to the rightmost ball, which flies up. This ball, in turn, will swing back down, imparting the energy and momentum in the other direction.
By analogy, an electron striking an ensemble of electrons from one side can influence an electron on the far side of the ensemble. The intervening ensemble is referred to as a Fermi sea since electrons are fermions, particles with a spin value of ½. And just as in the mechanical case one ball cannot penetrate the other balls but can impart energy and momentum to a ball on the far side, so one electron can smack into a Fermi sea and impart spin information to an electron on the far side. This transfer of quantum information across a Fermi sea is the heart of the proposed qubit-interaction plan.
In the JQI qubit scheme, repeated interactions between the spins in the two impurity atoms (corresponding to the outer balls of Newton's cradle) will be mediated by the spins in the quantum dot (which correspond to the balls in between). Of course, atoms and electrons are not mere balls. They possess charge and spin, and their interactions will still be a combination of electric and magnetic forces. And instead of gravity operating to power the slamming balls, electrostatic forces applied by electrodes at the surface of the sample are what keep the spins knocking away at each other.
Lake of electrons
A sprinkling of phosphorus atoms lies in crystal of silicon atoms, which provides a semiconducting environment for the atoms relatively free from disturbances. Just above the Si layer is a layer of silicon dioxide. In between the two layers is a two-dimensional sea of electrons (a Fermi sea) whose movements can be manipulated by the electrodes mentioned above. With just the right applied voltages the electrodes can pinch off regions of the lake, where only a few or even no electrons are allowed.
This gate-controlled pinching is how many transistors work in electronic devices like computers. It is also how the size and shape of quantum dots can be fashioned entirely by electrostatic forces. Those same electrodes manipulate the spins of the impurity atoms and the electrons in the quantum dot.
How many electrons are needed in the dot? The first author on the JQI paper, postdoc Vanita Srinivasa, was surprised to learn that the atom-dot scheme works with as few as two electrons in the dot. Communication between the two P atoms can consequently be thought of as a Newton's-cradle juggling of four electrons: the two operative electrons in the dot and the available electrons in the two impurity atoms.
Entanglement
To operate as a quantum computer, qubits have to be entangled. Here the entanglement of the two atomic spins—-making them part of a single quantum entity—-is brought about by a method analogous to the process by which the atomic qubits themselves are prepared.
When microwaves of the right frequency strike an atom, the atom can be excited from its ground state to a higher level. If the microwaves continue to be applied, the atom will be de-excited back to its ground state. The probability of the atom being in the excited state will vary sinusoidally with time. If the microwaves are carefully turned off at just the right moment (halfway through the first peak) the atom will have an equal likelihood of being in the ground and excited state. This is what makes the atom a qubit: it exists in two forms simultaneously.
Now consider the dynamic ensemble of four electrons in the JQI theoretical scheme. A proper sequence of voltage pulses can rock the atom-dot-atom ensemble back and forth in Newton's-cradle fashion, creating a corresponding flip-flop oscillation between the atomic spins. The rate of this oscillation can be tuned simply by adjusting the voltages that determine how easily electrons are able to (virtually) move between the atoms and the dot. If the flip-flopping is stopped at some point, the spin states of the two phosphorus atoms will be coordinated, while the state of the two dot electrons will remain separate.
In other words, the two P-atom qubits are entangled. Without necessarily knowing the exact status of the qubits, if some operation is performed on one of the qubits (such as flipping its spin state) the other qubit will undergo a comparable operation.
Two qubits entangled in this way represent a fundamental logic gate for building a quantum processor. The fidelity of this gate—-how well an operation on the qubits matches the desired operation —-can be optimized simply by tuning the voltages, which can lead to very high theoretical fidelities (up to 99.8%) even when electrical noise is taken into account and for relatively small interaction strengths.
"Fundamentally, the work suggests that a two-electron system represents the smallest possible Fermi sea," said Srinivasa. "By moving from one to just two electrons in the mediator dot, we find a more robust way to link the qubits. From a practical standpoint, current experiments on hybrid atom-dot systems suggest the exciting prospect of implementing dot-mediated atomic spin coupling in the near future."
More information: "Tunable Spin-Qubit Coupling Mediated by a Multielectron Quantum Dot." Phys. Rev. Lett. 114, 226803 – Published 4 June 2015. DOI: 10.1103/PhysRevLett.114.226803
Journal information: Physical Review Letters
Provided by Joint Quantum Institute