January 30, 2012 feature
The hidden nanoworld of ice crystals: Revealing the dynamic behavior of quasi-liquid layers
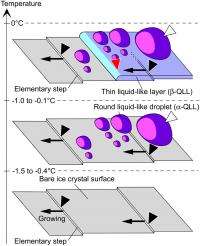
(PhysOrg.com) -- A wide range of phenomena depend on ice – specifically, phase transitions during ice crystal surface melting. In this transition, which occurs near the melting point, the ice surface morphs into what is known as a quasi-liquid layer (QLL) – a thin layer of ice grains where the water molecules are not in rigid solid structure, yet not in the random order of liquid. While previous research showed that QLL thickness increased with temperature, the results varied by as much as two orders of magnitude. Recently, however, scientists at Hokkaido University, in conjunction with Olympus Engineering Co., Ltd., developed a technique – laser confocal microscopy combined with differential interference contrast microscopy – that increases spatiotemporal resolution to point needed to visualize transition process of the 0.37nm (the thickness of one water molecule) step-like crystal surface at 0.1 – 4 seconds per frame. Their findings provide molecular-level understanding of QLLs that has implications for many QLL-dependent applications, including skating rink slipperiness, regelation (pressure-induced change in freezing), ice column frost heave, recrystallization and coarsening of ice grains, morphological change of snow crystals, cryopreservation, and electrification of thunderclouds.
Led by Professor Yoshinori Furukawa and Associate Professor Gen Sazaki at Hokkaido University’s the Institute of Low Temperature Science, the research team faced a number of challenges in uncovering the subnanometer structures and processes that held the key to understanding QLLs. “In 2004, I and Olympus Corporation developed an advanced optical microscope,” Sazaki told PhysOrg.oom, “by which we can visualize elementary steps – that is, the growing ends of ubiquitous molecular layers that are several nanometers in height – on protein crystals. We kept improving this microscope further, and finally succeeded in visualizing elementary 0.37 nm-thick steps on ice crystal surfaces. Since this optical microscope has ultimate sensitivity in the vertical direction, we could visualize surface melting processes of ice crystals for the first time.” Sazaki quickly acknowledges, however, that the appearance of QLLs on ice was first proposed by English physicist Michael Faraday in 1842.
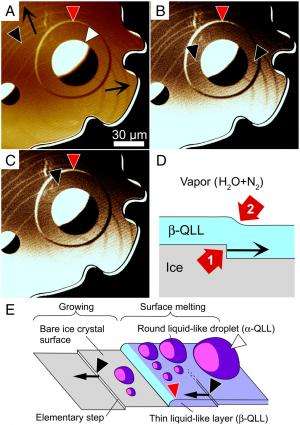
Sazaki also outlined the key insights and innovations the team used to address these challenges. “So far, it has been thought that only one type of quasi-liquid layer appear uniformly on ice crystal surfaces during the surface melting below 0 °C. However, we found that two types of QLL phases appear dynamically and spatially inhomogeneously. This result marked a sharp divergence from the previous view.” In addition, Sazaki adds that it’s quite interesting, from the fundamental scientific viewpoint, that two liquid phases made up of same water molecules are immiscible – that is, not forming a homogeneous mixture when added together.
Looking forward, Sazaki notes that surface-sensitive spectroscopy techniques, as well as scanning probe microscopy, will give the team a greater ability to understand the two QLL phases – in particular, how they differ. “We’re planning to develop a Raman microscope that, when combined with our present microscope, will allow us to measure the molecular motion of water. This will reveal how the two quasi-liquid layer phases are different.”
In terms of simulation, Sazaki points out that it will be difficult to fully model two QLL phases in silico, since still the physical and chemical properties of those phases are unknown. However, he adds, studies using molecular dynamics will also contribute significantly to our understanding of surface melting.
Regarding the impact of the team’s findings on the development of applications and technologies where surface melting-induced QLLs are a key factor, Sazaki told PhysOrg that it’s been believed that QLLs play important roles in the variety of phenomena mentioned above. “Since we can now directly visualize the surface melting processes, we can start to reveal the secrets of those phenomena."
In addition, Sazaki concludes, their research “will contribute to various fields such as meteorology, environmentology, cryobiology, material science, crystal growth physics, and other important areas.”
More information: Quasi-liquid layers on ice crystal surfaces are made up of two different phases. Published online before print January 9, 2012, PNAS January 24, 2012 vol. 109 no. 4 1052-1055, doi: 10.1073/pnas.1116685109
Copyright 2012 PhysOrg.com.
All rights reserved. This material may not be published, broadcast, rewritten or redistributed in whole or part without the express written permission of PhysOrg.com.