Part I: The energy that drives the stars comes closer to Earth
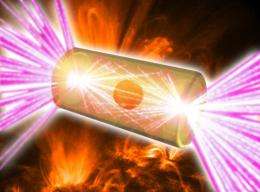
Nuclear fusion drives the stars, including our sun. But on Earth, despite efforts dating to the 1940s, sustained and controlled fusion for electrical power production has never been realized. Research persists, because few energy sources could be greener. Fusion yields far more energy than any other source per unit of mass; its heavy-hydrogen fuel is plentiful in sea water; burning it produces not a trace of carbon.
There’s more than one scheme for producing fusion power; the two main categories are magnetic confinement and inertial fusion energy. A promising approach to inertial fusion energy, called heavy-ion fusion (HIF), has long been advocated by scientists at the Department of Energy’s Lawrence Berkeley National Laboratory (Berkeley Lab).
In inertial fusion, the fuel is a solid target made of frozen isotopes of hydrogen (deuterium, or deuterium and tritium), which is instantly heated to fusion temperature when hit by driver beams of laser light or energetic particles. The heavy-ion fusion approached pursued at Berkeley Lab uses driver beams of ions (atoms lacking one or more electrons) whose atomic mass is generally greater than 100 – cesium or xenon, for example. (For comparison, iron has an atomic mass of 55.85.)
Most controlled-fusion efforts today involve magnetic confinement, however, with the most common reactors being the doughnut-shaped chambers called tokamaks. Tokamaks try to contain, squeeze, and heat a plasma of heavy hydrogen isotopes with magnetic fields, long enough for the nuclei to fuse. Magnetic-confinement research continues, but the inertial fusion energy approach has lately been gaining new attention.
Working toward heavy-ion fusion
In the spring of 2011, Berkeley Lab’s Accelerator and Fusion Research Division (AFRD) held a Workshop on Accelerators for Heavy Ion Fusion that drew 68 participants from national laboratories, universities, and private companies in the U.S., Germany, and Japan. For four days the members debated the best kinds of ion sources, the best kinds of ion-beam accelerators, the interface between the beams and the reactor chambers, and the best kinds of targets and how to position them.
“A couple of things are driving renewed interest in inertial fusion, and especially heavy-ion fusion,” says AFRD’s Peter Seidl, who chaired the workshop’s organizing committee. “One is the National Ignition Facility’s campaign to achieve ‘burn and gain,’ in which more energy is generated from the fusion reaction than went into creating it. Ignition is the sine qua non of fusion power, a goal they hope to reach soon.”
Although intended mainly for weapons research – by testing fusion reactions in miniature – the laser-based National Ignition Facility (NIF) at Lawrence Livermore National Laboratory has always had the important goal of demonstrating the potential of fusion power production as well. Successful “burn and gain” ignition is a necessary first step to validate the promise of inertial fusion energy.
NIF is a multi-billion dollar installation that uses 192 high-powered laser beams to ignite pea-sized targets inside a small gold cylinder called a hohlraum (German for “hollow space”). What ignites the target are not the driver laser beams themselves but pressure from the x-rays these beams produce when they bounce off the inner walls of the hohlraum.
“A second thing that’s sparked interest in heavy-ion fusion is that the National Academies will soon issue a report on the prospects for inertial confinement,” Seidl says. “Their mission is to evaluate the various systems, identify the challenges, and advise DOE on a research program that would deliver the scientific and technical basis for a decision to build an IFE demonstration plant.”
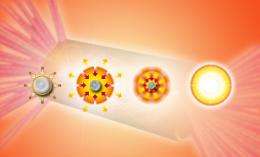
Seidl points out that such a facility would necessarily be on the scale of NIF – in other words, big – but devoted solely to energy research and the demonstration of power production.
Early designs for a heavy-ion fusion reactor called for high-power beams of ions, produced in an accelerator, to be focused on a pea-sized hohlraum and fusion capsule like those being tested at NIF. The ion beams would heat the hohlraum, in turn bathing the capsule in x-rays. This would ablate the surface of the fusion capsule inside, creating a rocket effect that would implode and crush it. The fuel would burn so quickly that it would be confined long enough to ignite and maintain fusion.
One advantage for heavy-ion fusion is the familiar technology of particle accelerators. Existing heavy-ion accelerators for basic research, like the Relativistic Heavy Ion Collider (RHIC) at Brookhaven National Laboratory or the Large Hadron Collider (LHC) at CERN (when used to collide heavy ions like lead instead of protons), have individually demonstrated several of the necessary features required by HIF.
These machines use radio-frequency (RF) power to create a series of strong electric fields that accelerate bunches of atomic nuclei to very high energies in two opposed beams. To generate the required power, however, HIF would require many more beams, accelerating many more ions per bunch.
HIF researchers choose a combination of ion energy and ion mass that allows a short “ion range” – short enough that the ions deposit virtually all their energy near the surface of the target. This is a much lower energy than the top energy at RHIC or LHC, suggesting that a different kind of accelerator is needed for optimum performance.
More information: Part II: The energy that drives the stars - different technologies for unique demands
Provided by Lawrence Berkeley National Laboratory