Explained: Bandgap
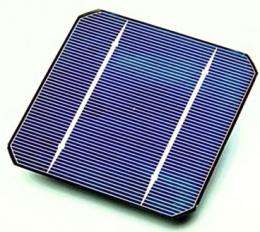
Why do some materials work well for making solar cells or light-emitting diodes (LEDs), while other materials don't? One key factor is having the right bandgap.
In a nutshell, bandgaps have to do with how electrons behave and what it takes to get them excited. Electrons are the subatomic particles that carry a negative charge, and that surround the nucleus of an atom. When a bunch of electrons all move together in the same direction, they form an electric current.
Electrons in an atom can be thought of as being somewhere in an array of possible “states” — which include their energy level, momentum and spin — with different probabilities of being in a given state. Two electrons can’t be in the same state at the same time — that is, at least one of these variables must differ. Some particular states are possible, and some are forbidden by the laws of quantum mechanics. Sets of possible states form regions that are called bands. Sets of states that are not possible form regions between those bands, and these are called bandgaps.
The bands closest to the atomic nucleus, called core levels, and the furthest band from the nucleus that has electrons in it, called the valence band, all keep their electrons tightly in place. The next band out from that is called the “conduction band,” and there, the electrons are free to roam around freely.
In some materials, called metals, a valence band and a conduction band overlap, and electricity flows freely and easily through them. In other materials, called insulators, there is a wide gap between the valence band and the conduction band, making it almost impossible for an electron to get excited enough to jump from one to the other, so they block the flow of electricity.
There’s a third category, and that’s where the most interesting stuff happens. These are materials that have a narrower gap between the two bands, and they are called semiconductors. Sometimes they can act like metals, sometimes they can act like insulators, and sometimes they can have properties in between. When first discovered, they were considered useless because of their erratic, variable behavior — until physicists figured out the mystery of the bandgap.
“It was the idea of the bandgap that allowed people to understand and harness semiconductors for optoelectronic devices” — that is, devices that work with light and electricity — says Tonio Buonassisi, MIT’s SMA Assistant Professor of Mechanical Engineering and Manufacturing.
When electrons get excited (by getting heated, or by being hit with a particle of light, known as a photon), they can jump across the gap. If an electron in a crystal gets hit by a photon that has enough energy, it can get excited enough to jump from the valence band to the conduction band, where it is free to form part of an electric current. That’s what happens when light strikes a solar cell, producing a flow of electrons.
Silicon, a semiconductor, is the material of choice for solar cells in large part because of its bandgap. Silicon’s bandgap is just wide enough so that electrons can easily cross it once they are hit by photons of visible light.
The same process also works in reverse. When electricity passes through a semiconductor, it can emit a photon, whose color is determined by the material’s bandgap. That’s the basis for light-emitting diodes, which are increasingly being used for displays and computer screens, and are seen as the ultimate low-power light bulbs.
More information:
Video: "Next Generation Solar Cells" with Tonio Buonassisi - mitworld.mit.edu/video/675
More "Explained" stories: www.physorg.com/search/sort/da … xplained:&headline=1
Provided by Massachusetts Institute of Technology