August 29, 2014 feature
Step lightly: All-optical transistor triggered by single photon promises advances in quantum applications
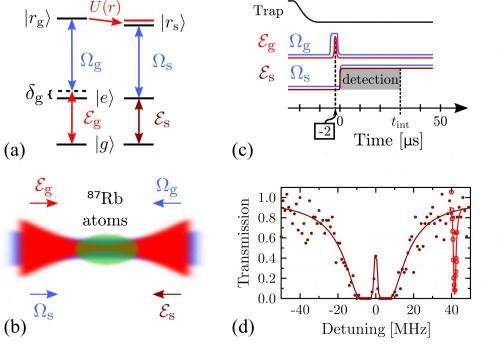
(Phys.org) —Optical transistors and switches are fundamental in both classical and quantum optical information processing. A key objective in optics research is determining and developing the structural and performance limits of such all-optical devices, in which a single gate photon modifies the transmission or phase accumulation of multiple source photons – a feature necessitating strong interaction between individual photons. While significant progress has been made – especially in cavity QED experiments, which use resonators to enhance interaction between photons, confined in a reflective enclosure, and natural or artificial atoms – the goal is to achieve high optical gain and high efficiency using a free-space – that is, cavity-free – approach. Recently, scientists at Universität Stuttgart, Germany demonstrated a free-space single-photon transistor based on two-color Rydberg interaction, which they say could lead to a high optical gain, high efficiency optical transistor through further improvements. (In a Rydberg atom a single electron is excited to a state with a large principal quantum number, meaning that it has high potential energy.) Moreover, the researchers state that the finding may lead to advances in quantum information processing, condensed matter physics, single step multi-photon entanglement, and other important areas.
Doctoral students Hannes Gorniaczyk and Christoph Tresp, along with Dr. Sebastian Hofferberth, discussed the paper that they and their co-authors published in Physical Review Letters. They first addressed the challenge of devising a novel approach to implement a free-space single-photon all-optical transistor with an optical gain exceeding a factor of 10. "Photons do not inherently interact," Gorniaczyk tells Phys.org. "Therefore, one has to think about ways of introducing interaction – and it's been shown that the interaction of Rydberg atoms in a cold atomic cloud can be mapped onto the photons to create an effective interaction."
"Due to the large micrometer size of these atoms," Tresp points out, "the interaction between them is very strong – and we use this property to create strong interaction between single photons. The new aspect of our transistor scheme is that we use two different Rydberg states at the same time, allowing us to independently address the gate and source photons." This approach enables the scientists to show that an optical gain G > 10 can be reached with, on average, less than one gate photon.
Another challenge was mapping gate and source photons into Rydberg excitations with different principal quantum numbers in an ultra-cold atomic ensemble. "To map the gate and source photons of the optical transistor into Rydberg excitations requires experimental effort." Gorniaczyk explains. "First, it's necessary to create a cold and dense cloud of atoms in a favorable geometry; then, four excitation laser beams have to be overlapped and aligned on the atomic cloud; and finally, while the strong interaction between Rydberg atoms is the key to our scheme, they are also very susceptible to any external perturbation, requiring great care in shielding the experiment from external electric fields."
A key result was using the optical transistor to demonstrate the nondestructive detection of a single Rydberg atom. The conventional method is to ionize Rydberg atom and detect the resulting ion on an ion detector, with typical detection efficiencies below 0.5. However, by using their optical transistor the researchers mapped the existence of one Rydberg atom onto a probe light pulse by over 10 photons – which would be impossible if source photons destroyed gate excitations. This makes the new approach well-suited to independent control of gate and source photons. "While this is very similar to how electronic transistors are used to amplify signals," Tresp notes, "our new approach is a clear improvement: Even with all imperfections taken into account, we achieve fidelity of 0.72 for the single shot detection of a Rydberg atom without destroying it during the process." Moreover, optical transistors based on the interaction of Rydberg excitations are robust, and photon Interaction can be mediated by interaction between Rydberg states with different principal quantum numbers.
In addition, the paper notes that their novel approach could enable a high optical gain, high efficiency optical transistor, which so far has been realized only in a cavity QED setup, in which attenuation of hundreds of source photons has been demonstrated. "Cavity QED experiments employ an optical resonator to increase light-matter interaction," Gorniaczyk says. "However, resonators come with technological challenges and fundamental limitations, such as limited bandwidth, which free-space experiments do not suffer."
"Optical transistors open a wide range of potential optics applications, one of which is the single-shot detection of single photons," Hofferberth tells Phys.org. "In addition, investigating different state combinations can lead to an increase in optical gain. In particular, the use of so-called Förster resonances1, as well as working with states that are directly coupled, can significantly boost performance. Modification of trap geometry for the cold atomic cloud would also improve our system by leading to a greater optical depth for source photons in the presence of gate photons – and thus to a higher attenuation. Finally, the retrieval of gate photons after the transistor experiment would open up the door to quantum computation."
Furthermore, the paper points out that the combination of two independently controlled Rydberg-EIT (electromagnetically induced transparency) schemes offers high flexibility. With two excitation schemes, the gate photon can efficiently be stored using a detuning to the intermediate state, whereas the source photons can propagate through the medium with reduced group velocity. Additionally, the two-color scheme enables novel fields of study, such as Förster resonances or directly dipole-dipole coupled Rydberg states. "Förster resonances occur when a pair state involving two Rydberg states resonantly couples to another pair state," Tresp explains. "Using a suitable resonance allows to increase the interaction between gate and source photons, while the interaction among source photons can be kept low. This can lead to an increase in gain."
Theoretically, the researchers say, their optical transistor can be extended such that an imaging system with source photons can determine the position of gate excitations in a single shot. If the interaction between gate and source photons is very high, the exact position of gate excitations cannot be tracked back, so the resolution decreases with increasing interaction, especially if more gate excitations are present. "Moreover, our optical transistor can be seen as a device that entangles the gate photon with many source photons," Gorniaczyk says. "The presence of source photons, for instance, implies the absence of the gate photon in the first place. Systems with strong many-body correlations are of high interest in quantum information technology, and many ideas could be realized including quantum gate arrays." Quantum gate arrays are basic quantum circuits that process small numbers of qubits and are combined to construct quantum circuits.
Another possible quantum computation application is the experimental embodiment of a two-photon phase gate based on Rydberg-polariton collisions(Polaritons are part matter, part light quasiparticles).) "We currently show that a gate photon efficiently attenuates source photons –or in other words, the gate photon causes absorption of source photons," Hofferberth tells Phys.org. "It's also possible, though, to modify the scheme such that the gate photon causes dispersion of the source photons, causing a phase shift of the source photons. The only modification required for this is to send the source photons with a different detuning into the medium. Currently they are tuned exactly onto resonance with an optical transition to cause strong absorption, but by detuning them the effect can be turned into a conditional phase shift." A two-qubit phase-gate is a universal quantum gate, meaning that if a reliable phase gate is available along with single qubit gates), any quantum computation can be realized. "Our system would be the first purely photonic deterministic two qubit gate," Hofferberth adds.
In addition to the research plans discussed above, Gorniaczyk says since their work demonstrates controlled interaction between individual photons , the scientists can now turn towards realizing long-standing goals such as all-optical quantum computation. "Our explicit next goal is to demonstrate that the transistor can be operated with gain greater than 1, and the gate photon retrieved afterwards with all of its quantum properties intact. This will enable multi-photon entanglement in a single step and entangled states of multiple particles – in this case, the latter being photons – which are a key quantum information resource."
Another of their ideas is similar to the non-destructive detection of single atoms: If the gate photon can survive the transistor process, it will enable non-destructive quantum state detection of single photons. "Currently, all photon detection methods are destructive and limited in efficiency – in practice, usually to below 0.3," Tresp explains. "A non-destructive detector with high fidelity would be an innovation with many applications in optical science."
Addressing other areas of research that might benefit from their study, Hofferberth notes that while their current work focuses on making the Rydberg-mediated interaction between photons useful for quantum applications, more generally it realizes a novel system of strongly interacting particles. "If the number of photons interacting with each other simultaneously can be further increased, such a system could be a novel approach to study many-body physics problems, as encountered in condensed matter physics. In addition," he concludes, "the enormous flexibility of our system could be the foundation of a photon-based quantum simulator used to investigate systems that cannot easily be studied directly."
More information: Single-Photon Transistor Mediated by Interstate Rydberg Interactions, Physical Review Letters 113, 053601 (28 July 2014), doi:10.1103/PhysRevLett.113.053601
Related:
1Single-Photon Transistor Using a Förster Resonance, Physical Review Letters 113, 053602 (28 July 2014), doi:10.1103/PhysRevLett.113.053602
Journal information: Physical Review Letters
© 2014 Phys.org