On-chip quantum buffer realized
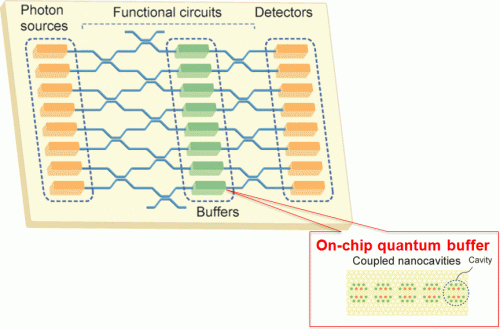
Nippon Telegraph and Telephone Corp. has realized a quantum buffer integrated on an optical waveguide. The buffer is based on the "slow light effect", where the propagation speed of a pulsed light in a special optical waveguide slows significantly compared with the speed of light in vacuum.
The buffer facilitates the precise synchronization of photons in two photon quantum interference experiments needed for realizing quantum gates, and thus moves us forward in our goal of realizing quantum computers based on photonic quantum bit (qubit).
This result will be published in the UK science journal Nature Communications on November 12, 2013.
There are many schemes for the physical realization of a qubit that can be used for quantum computing, including superconducting devices, semiconductor nanostructures, atoms and photons. Among them, a photon can be used as a stable qubit, since a photon rarely is affected by noise in environment.
To construct a quantum computer, we need "two-qubit quantum gates" where logic operations to two qubits are undertaken by interacting them. In the case of photonic qubits, it is well known that such gates are constructed using the quantum interference of photons. Recently, intensive research has been undertaken to build integrated quantum optical circuits in order to enable and realize large-scale photonic quantum computers.
For the realization of quantum gates based on photon interference, we need to synchronize the arrival times of photons at the photonic quantum circuits very precisely. A quantum buffer, where a photon is stored while faithfully preserving its quantum state, can facilitate the synchronization of the photons and thus achieve a quantum gate operation.
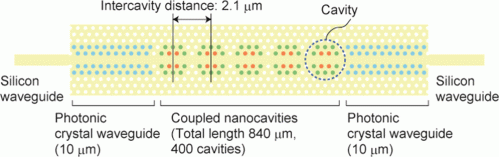
We have realized a quantum buffer by using the "slow light effect" (where the speed of pulsed light is significantly reduced compared with the speed of light in vacuum) in coupled nanocavities fabricated utilizing our silicon photonic crystal fabrication technology.
We undertook our experiments described below to evaluate the performance of these coupled nanocavities as a quantum buffer. As a result, we successfully demonstrated that the buffer could slow down the speed of pulsed photons to 1/60 of the speed of light in vacuum while faithfully preserving its quantum state. This result confirmed that the developed device could be used as a quantum buffer integrated on chip.
The use of the quantum buffer will facilitate the synchronization of photons in various patterns of optical circuits, and thus is expected to increase the integration level of the quantum optical circuit. In addition, the tunability of our buffer will help realize the reconfigurable quantum optical circuit.
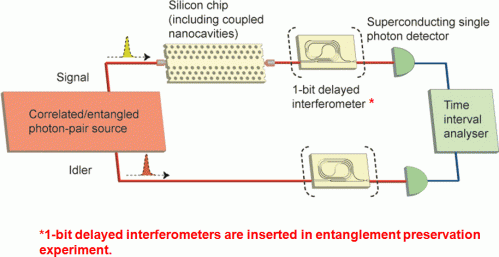
- A photon from a quantum correlated photon pair source was transmitted through the silicon photonic crystal coupled nanocavities, and the temporal correlation between the two photons was measured. As a result, we confirmed that the photon was stored in the coupled nanocavities for 150 ps while the temporal correlation between the two photons was preserved.
- We confirmed that the delay time could be tuned by 50 ps by changing the chip temperature.
- Further, we showed that the buffer preserves entanglement by storing and retrieving one photon from an entangled photon pair.
Technical Features:
- Silicon photonic crystal coupled nanocavities: Since an optical cavity has an ability to store light, we can obtain the slow light effect by coupling many cavities in an array. NTT Basic Research Laboratories fabricated coupled nanocavities with a total length of 840 μm using the silicon photonic crystal technologies. The number of cavities was 400, which is the largest number of coupled nanocavities ever reported. The use of this device made it possible to reduce the propagation speed of a pulsed light to 1/60 of the speed of light in vacuum while preserving the pulses waveform.
- Photon delay measurement using telecommunication-band quantum correlated/entangled photon pairs: The coupled nanocavities used in the experiments can work as a slow light medium only for 1.5-μm telecommunication band photons. Therefore, we generated 1.5-μm quantum correlated/entangled photon pairs via spontaneous four-wave mixing in an optical fiber and used them for the delay observation and entanglement preservation measurements. We also used superconducting single photon detectors by which we could detect pulsed photons with high signal-to-noise ratio and high temporal resolution. The use of quantum optical measurement techniques in the 1.5-μm band developed by NTT Basic Research Laboratories contributed to the success of high-resolution temporal delay measurement of single photons and confirmation of entanglement preservation.
We will develop high-efficiency single photon detector integrated on a chip. Then, we will build an integrated quantum optical circuit where components such as photon sources, detectors and buffers are implemented on a chip for realizing photonic quantum computers.
More information: Hiroki Takesue, Nobuyuki Matsuda, Eiichi Kuramochi, William J. Munro, and Masaya Notomi, "An on-chip coupled resonator optical waveguide single-photon buffer, "Nature Communications (2013)
Journal information: Nature Communications
Provided by NTT