Determining the quantum geometry of a crystal
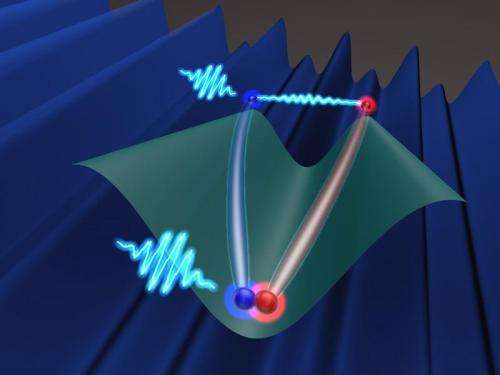
Geometrical phases occur in many places in nature. One of the simplest examples is the Foucault pendulum: a tall pendulum free to swing in any vertical plane. Due to the earth rotation, the actual plane of swing rotates relative to the earth. One observes that every day the plane of rotation changes by a small "geometric" angle, associated to the spherical shape of the earth. In quantum mechanics a similar effect was discovered in 1984 by the British physicist Sir Michael Berry, who identified a geometrical phase in quantum-mechanical problems that is today known as the "Berry's phase". Such quantum-mechanical phases can have a profound effect on material properties and are responsible for a variety of phenomena. Some examples are the dielectric polarization or the quantum Hall effect, with the latter one being used nowadays to define the standard of resistance.
For the first time, scientists in the group of Professor Immanuel Bloch (Ludwig-Maximilians-University, Munich and Max-Planck-Institute of Quantum Optics, Garching) in close collaboration with theoretical physicists from Harvard University in the group of Professor Eugene Demler have succeeded in measuring such a phase in a one dimensional solid-state like system. This phase is known as the "Zak-phase" named after the Israeli physicist Joshua Zak.
Two objects have a different topological structure if there is no continuous way to transform one to the other without cutting it or punching holes in it. For example, a tee cup with a single hole in the handle and a bagel are topologically equivalent, but a bagel and a soccer ball are not. Furthermore, the different topological structures can be characterized by the geometric phases associated with the shape of the object. But, what do these geometric phases have to do with the properties of a real material? "In a material, the atoms are organized forming a periodic structure where the electrons experience the electric forces of the ions. As a result, the electrons "move" inside the material in so-called energy bands, which play the role of the objects in the examples above," explains Marcos Atala, a senior PhD student at the experiment.
In 1989, Israeli Physicist Joshua Zak identified the geometrical phases in the band theory of one-dimensional solids: when a particle travels "slowly" across the energy band and completes a closed loop, it acquires a geometrical phase that has striking physical consequences for the properties of materials: light transmission, electrical conduction, or response to a magnetic field can all be determined by the "quantum geometry" of the crystal. Therefore the identification of the topological properties of a band is fundamental for understanding its physical properties.
In their experiments, an extremely cold gas of rubidium atoms was loaded into an optical lattice: a periodic structure of bright and dark areas, created by the interference of counter-propagating laser beams. In this lattice structure, the atoms are held in either dark or bright spots, depending on the wavelength of the light, and therefore align themselves in a regular pattern. The resulting periodic structure of light resembles the geometry of simple solid state crystals where the atoms play the role of the electrons. The use of an additional light field with twice the spatial period allowed the scientists to create an optical superlattice in which the periodic structure has a regular pattern of low- and high-energy barriers similar to a polyacetylene molecule, which possesses rich topological properties.
In order to measure the Zak phase, the Munich researchers implemented a protocol proposed by the team of their Harvard colleagues in the group of Eugene Demler. The measurement idea used by the team is in fact closely related to the working principle of an optical interferometer. There, a light beam is split and allowed to propagate along two paths. Recombining and overlapping the two beams leads to an interference pattern in which the phase of the resulting interference stripes is determined by the phases acquired by the light waves during propagation along the two paths. Taking advantage of the laws of quantum-mechanics that allow a single particle to be in two states at the same time, in their experiments the researchers prepare the system in a superposition of spin up and spin down. A force, which depends on the spin state of the atom, is then applied such that the two components of the atom explore the energy band in different directions (See Fig. 1). During their motion through the band, the particles pick up the Zak phase that is determined by the quantum geometry of the band. Similar to the optical interferometer, interfering the two spin components of the atom, the researchers were directly able to reveal the geometric phase of the crystal.
Previously, the measurement of geometric phases in solids could only be carried out indirectly, and required a filled energy band for the measurement. With this new method, only single particles are required. These need to be transported gently through the energy band such that they can explore the underlying quantum geometry of the crystal. Eugene Demler and his team also pointed out simple generalizations of this scheme to higher dimensions or even problems including many interacting particles. "This new measurement scheme establishes a new general approach for studying the topological structure of Bloch bands in solids," points out Immanuel Bloch. The new experimental probes might thus lead to the discovery of novel topological phases of quantum matter with unique properties that may be useful for practical applications.
More information: Marcos Atala, Monika Aidelsburger, Julio T. Barreiro, Dmitry Abanin, Takuya Kitagawa, Eugene Demler and Immanuel Bloch, Direct measurement of the Zak phase in topological Bloch bands, Nature Physics, AOP DOI: 10.1038/nphys2790
Journal information: Nature Physics
Provided by Ludwig Maximilian University of Munich