Smaller than small: Why we measure the space between atoms
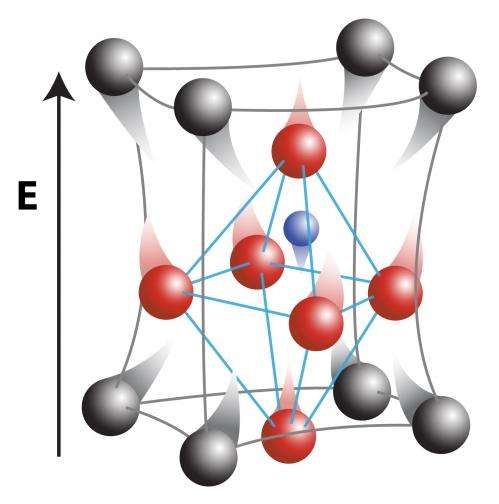
We study the movement of incredibly small things. How small is small? Think smaller than "nano." Think smaller than atoms themselves. We measure the infinitesimally small shifts in the positions of atoms to electrical forces. Measuring small is challenging, but rewarding. By measuring things this small, we unlock hidden secrets that will advance a host of different electronic devices.
How and why? Let's start with the basics.
Most people know that metals are good at conducting electricity. That means that electrons can move long distances through most metals. The electrical power grid is a perfect example of this fundamental material behavior in action and is one of the most recognizable applications of electrical conductivity.
In contrast, insulating materials are those in which this effect is reduced by 10-20 orders of magnitude. Effectively, electrons can hardly move at all in insulating materials. Since these materials don't (usually) allow electrons to move, some of their most basic applications are to protect and direct electrical conductors. Think of the protective coating around a power cord.
Electrons are still fundamentally important to insulating materials, but play a different role. Before being pushed by an electrical force, electrons are strongly bound to certain atoms, giving rise to positively charged "cations" and negatively charged "anions." When pushed using electrical forces (like voltages), the cations and anions can move ever so slightly. The picture above is an exaggerated illustration of these very small movements.
The distance between these cations and anions is small to start with – measuring near 10-10 meters, or smaller than the nanometer scale. And the changes in their positions during applied voltages are even smaller than small – measuring 10-15 to 10-17 meters! Yet those small displacements are essential to a number of high-tech applications, from microelectromechanical (MEMS) systems to high precision control of mirrors for optics and satellite systems.
One of the challenges in our research community is how to measure something so incredibly small. The optical microscope is limited to resolving features such as biological cells – much too large for resolving atoms and small atom movements. I'm leading a team of researchers at NC State that's using high-energy X-rays to measure these effects. The wavelength of these X-rays, on the order of 10-10 meters, can be used to measure the almost infinitesimal distances between atoms. And specialized equipment and thorough analysis of measured signals can presently reveal changes in atomic movement down to nearly 10-16 meters. This means that we measure some of these important atomic effects.
Once my team understands how the different cations and anions move under electrical forces, the research community can use that information to design better energy storage and conversion devices, such as capacitors, actuators, and piezoelectrics. We can finally begin from the bottom up and design these insulating materials starting at the atomic level. Presently, there is also emerging a multi-investigator, multi-university center of research on these materials at NC State, the Center for Dielectrics and Piezoelectrics, so the timing of these measurements will be useful to a number of related research projects.
More information: This is a guest post by Jacob Jones, an associate professor of materials science and engineering at NC State.
Provided by North Carolina State University