April 17, 2008 feature
The Ultimate Test of Atom and Neutron Neutrality
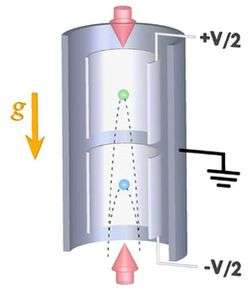
Researchers from Stanford University have proposed a new way to test the neutrality of an atom and even a neutron, a method they say will be far more sensitive than current methods, able to probe the charge of an atom or neutron down to an unbelievably small fraction of the electron charge, e.
This experiment will help answer important questions about charge quantization. Why does the charge appear in units of the electron charge? Why do some particles have no charge? Do the electrons and protons in an atom really cancel out to zero charge?
These questions are not answered by the Standard Model, the established theory describing elementary particles, their properties, and how they relate to each other. The Stanford group states in their paper describing the experiment, published in the March 27, 2008, online edition of Physical Review Letters, that an alternative “Grand Unified” theory (“grand” because it links together the electromagnetic, strong nuclear, and weak nuclear forces into one neat package) can automatically explain charge quantization and atom neutrality.
Even so, they quickly point out that a magnetic monopole, a theoretical particle with only one magnetic pole (instead of two like a bar magnet), has been predicted to be able to carry an amount of non-quantized electric charge, under violations of the time reversal symmetry in the theory.
“This suggests that we have to rethink our notion of charge quantization and the conditions under which it holds,” said Stanford physicist Asimina Arvanitaki, the paper's corresponding author, to PhysOrg.com. Arvanitaki is also affiliated with the Stanford Linear Accelerator Center.
Arvanitaki and her colleagues expect that their experiment can greatly improve the sensitivity by which the charge on an atom can be measured: from 10-20e to 10-28e—in other words, a hundred million times better.
“This is similar to measuring the distance between the earth and the sun to an accuracy better than the size of a nucleus,” said Arvanitaki.
The experimental setup is a type of atom interferometer, an instrument designed to detect tiny differences between atoms by exploiting the wave nature of an atom—the phenomenon that a particle, like a light wave, has its own wavelength, described by a mathematical function called a wavefunction, as predicted by quantum mechanics.
The Stanford setup consists of a large vertical cylinder 10 meters tall with two smaller cylinders inside, also oriented vertically but with a gap between them. At the bottom of the device, rubidium atoms are launched vertically into the lower small cylinder with an initial velocity of 10 meters per second (m/s).
As the atoms stream upwards, a series of meticulously timed laser pulses act on the atoms like mirrors and beam-splitters, breaking up each atom's wavefunction into a superposition of two space-time trajectories with a difference in momentum. This results in a fast-moving wavefunction component and a slower component, which later recombine and interfere.
When “large momentum transfer” beamsplitting is realized, it can lead to a difference in momentum that corresponds to a velocity difference of 1 m/s. This means one component will go significantly higher than the other: The parabola-shaped trajectory of the fast component will extend into the upper of the two small cylinders, while the trajectory of the slow component will not leave the lower cylinder.
Taking advantage of this, the researchers can then introduce two regions of electric potential in the device: a positive voltage applied to the upper cylinder and a negative voltage applied to the lower. If the rubidium atom carries a small charge, the wavefunction components will react to the voltage in their respective cylinders, and a phase shift will be introduced between them, meaning one component will oscillate more times than the other.
This difference can be measurable. When the experiment is repeated a million times and for a million atoms at a time, the statistics are improved and even charges per nucleon of 10-28e can be detected.
The researchers discuss increasing the number of atoms to between 10 and 100 million or using entangled states of atoms, another technique that takes advantage of the quantum mechanical properties of atoms. These changes, they say, can lead to an even higher sensitivity of 10-30e.
Citation: Phys. Rev. Lett. 100, 120407 (2008)
Copyright 2008 PhysOrg.com.
All rights reserved. This material may not be published, broadcast, rewritten or redistributed in whole or part without the express written permission of PhysOrg.com.