March 15, 2023 feature
This article has been reviewed according to Science X's editorial process and policies. Editors have highlighted the following attributes while ensuring the content's credibility:
fact-checked
peer-reviewed publication
trusted source
proofread
The experimental observation of quantum avalanches in a many-body localized system
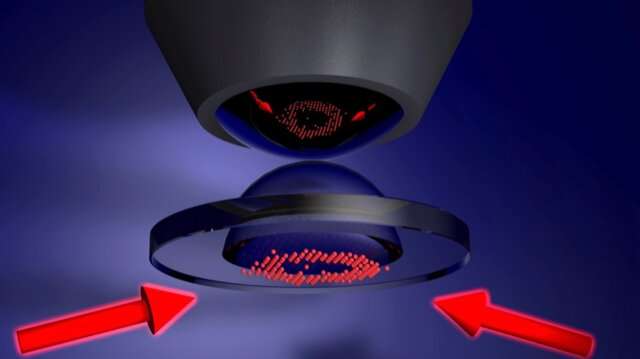
Strongly correlated systems are systems made of particles that strongly interact with one another, to such an extent that their individual behavior depends on the behavior of all other particles in the system. In states that are far from equilibrium, these systems can sometimes give rise to fascinating and unexpected physical phenomena, such as many-body localization.
Many-body localization occurs when a system made of interacting particles fails to reach thermal equilibrium even at high temperatures. In many-body localized systems, particles thus remain in a state of non-equilibrium for long periods of time, even when a lot of energy is flowing through them.
Theoretical predictions suggest that the instability of the many-body localized phase is caused by small thermal inclusions in the strongly interacting system that act as a bath. These inclusions prompt the delocalization of the entire system, through a mechanism that is known as avalanche propagation.
Researchers in the group of Markus Greiner at Harvard University recently carried out a study exploring this fascinating but so far experimentally elusive mechanism. Their study, featured in Nature Physics, led to the first experimental observation of the onset of quantum avalanches in a many-body localized system.
"Whether particles remain localized in a disordered potential, or whether they spread out, is a long-standing question that has occupied physicists for many decades," Julian Léonard, one of the researchers who carried out the study, told Phys.org. "This question is important because in materials, localization is linked to electronic transport, hence understanding the conditions when particles localize will tell us why certain materials are insulators or conductors."
The localization of particles is a quantum mechanical effect, as it relies on the wave nature of electrons and on the mechanism of entanglement (i.e., a quantum mechanical process through which particles, in this case electrons, become highly correlated). Gaining a better understanding of localization is a key objective for the physics community, as it could greatly inform both research and technology development.
Firstly, perfect localization is an interesting research topic because it contradicts thermodynamics, one of the most renowned and well-established physics theories. Secondly, a system with perfectly localized particles would be able to store quantum information for longer periods of time, thus understanding its underlying mechanisms could advance the development of quantum technology, particularly quantum memories.
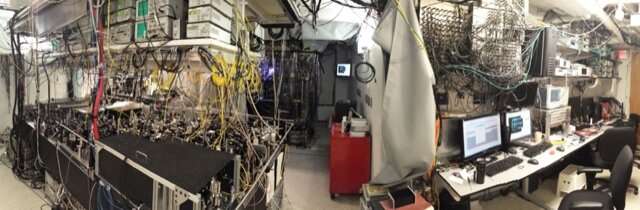
"These so-called quantum memories are necessary for quantum computing and communication protocols," Léonard said. "Several research groups, including ours, had previously seen that interacting particles can indeed localize, and there has been widespread consensus that this localization should prevail indefinitely. However, recently the robustness of localization has been debated, particularly what would happen if the disorder is a little bit weaker somewhere in the system. Could this be enough to destroy localization?"
The key objective of the recent study by Léonard and his colleagues was to closely examine localization and its robustness. Past theoretical calculations predicted that localization could be destroyed in an intricate and fascinating scenario.
Specifically, theorists predicted that under the right conditions, particles in a weakly disordered region could rapidly shift towards the strongly disordered part of a system, delocalizing it. This phenomenon is known as a quantum avalanche, as it can be seen as a wave of localized particles shifting towards this delocalized region, rapidly accelerating and delocalizing the whole system, resembling an avalanche.
"For us, the challenge was to realize such a system experimentally in the lab," Léonard said. "To do this, we placed cold atoms in a potential that we built out of precisely shaped laser beams. One part of the potential was disordered, the other part was without disorder. We then waited to see how these two regions would interact over time, and measured how far the particles would spread. With cold atoms, this can be done extremely well by observing them with an optical microscope."
Interestingly, Léonard and his colleagues found that initially the particles in the disordered part of their system would localize. Gradually, however, particles from the non-disordered region started to spread to the disordered one at an increasing speed, as theoretical predictions suggested they would.
These observations suggest that they successfully probed the onset of a quantum avalanche in an experimental setting for the first time. Notably, this could mean that localization is not as robust as it was previously believed to be and that it may not hold for very long times. These interesting findings could soon inspire new experiments aimed at further probing quantum avalanches and assessing the robustness of localization in strongly interacting many-body systems.
"Our experiments mark the discovery of quantum avalanches, but they are just the beginning of exploring their properties," Léonard added. "Many questions remain open, particularly under which conditions these avalanches occur, how often they emerge, and whether there could be ways to stop their propagation. These factors will ultimately determine whether localization is always unstable, or just for certain conditions. We are currently working on realizing systems with more atoms, where these questions could be studied in more detail."
More information: Julian Léonard et al, Probing the onset of quantum avalanches in a many-body localized system, Nature Physics (2023). DOI: 10.1038/s41567-022-01887-3
Journal information: Nature Physics
© 2023 Science X Network