Antimatter unveils the secrets of liquid crystals
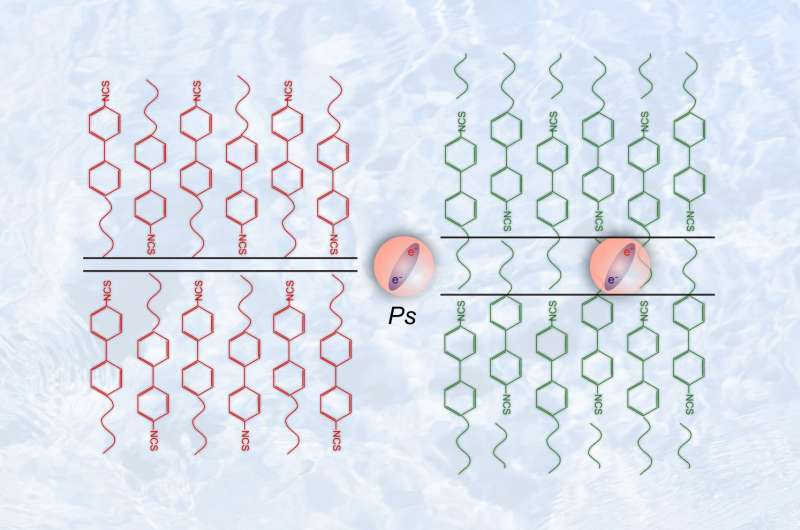
Liquid crystals combine such contradictory features as the chaos of liquid molecules, and the ordering characteristics of crystals. Thanks to an innovative application of antimatter, it has been demonstrated at the Institute of Nuclear Physics of the Polish Academy of Sciences in Krakow that the structures formed by certain molecules of liquid crystals must in fact be different than previously thought.
Liquid crystals are found in many fields of technology, and in the future, their use will likely grow, for example, as organic semiconductors. But to make this possible, basic research is needed using a variety of experimental techniques in order to reveal the structure of these compounds and their dynamics. To this end, researchers have conducted new experiments on the quenched smectic-E (Sm-E) phase of liquid crystals. Smectics of this type are composed of well-ordered particles separated into layers. Until recently, it was thought that the distance between the individual layers of particles was very small. Research conducted by the Krakow physicists helped to verify the correctness of current models and precisely determine the crystal-like phase structure.
"We have employed an interesting measurement technique rarely used in the case of liquid crystals. The method uses the specific characteristics of positrons, which are antimatter counterparts of ordinary electrons," explains Dr. Ewa Dryzek (IFJ PAN).
A positron as the antiparticle of an electron has a positive charge. When a positron meets an electron it may lead to annihilation, where the mass of both particles converts into electromagnetic radiation with a characteristic energy.
"In the world of ordinary matter, antimatter is produced by physical processes only in trace amounts. In the course of our measurements, we used positrons created in radioactive decay of the isotope sodium 22," says Dr. Ewa Juszynska-Galazka (IFJ PAN).
Positrons of a radioactive source penetrated the test material, in which they encountered electrons. Before the annihilation of a positron and an electron pair, an exotic atom called positronium can form. In soft matter such as liquid crystals or polymers, positronium may be formed in nanopores, which are small voids between molecules. Measuring its lifetime—the time between the emission of a positron from a radioactive source and its annihilation—allows us to determine the size of the nanopores. The smaller the nanopores, the faster the annihilation occurs.
Research at IFJ PAN focused on positron irradiation of a compound called 4TCB, which unlike many other substances, does not crystallize with a decrease in temperature, but with an increase. The results showed that in this material, positronium is formed. However, given the existing model arrangement of molecules in the Sm-E phase, it was difficult to identify the place where it could be accommodated.
"Our measurements show that positronium's nanopores are the size of approximately six angstroms, that is, six 10-billionths of a meter. These results were consistent with one of the variants of the new model of Sm-E, which only recently has been proposed by Prof. Kazuya Saito's group from Japan," says Dr. Dryzek.
Measurements have confirmed that the alkyl chains—the 'tail' of molecules—are in a liquid state, and thus have freedom of movement, as in an isotropic liquid. It is worth mentioning that in liquids, as a result of interaction with the surrounding molecules, the positronium repulses the neighbouring molecules or their parts to produce a small empty space around itself. Such an arrangement can be imagined as a bubble with positronium in its center.
The Japanese Sm-E model, proposed on the basis of calorimetric tests and the diffraction, assumed that the liquid crystal molecules are arranged in two layers—the first comprised of rigid phenyl rings, the other of alkyl chains.
"At this point, all the information began to fit together. Positronium can produce a bubble in the layer containing the alkyl tails, as they are in the liquid state. The size of the resulting bubble corresponds to the width of the layer," says Dr. Dryzek.
Temperature measurements of the positronium lifetime confirmed that at low temperatures, quenched 4TCB creates glass, in which the positronium cannot form. The movements of the alkyl tails are frozen and positronium cannot produce a bubble. With an increase in temperature, the glass softens, which can be described as the formation of liquid-like domains. It is in these domains that positronium begins to form.
Positron annihilation spectroscopy is used in the material testing of metals, semiconductors and polymers. The results of IFJ PAN prove that when applied skilfully, this method can be a source of intriguing and detailed information about the structure of liquid crystals.
More information: E. Dryzek et al, Positronium formation and annihilation in liquid crystalline smectic-phase revisited, Physical Review E (2016). DOI: 10.1103/PhysRevE.93.022705
Journal information: Physical Review E
Provided by Polish Academy of Sciences