Moon is younger than first thought
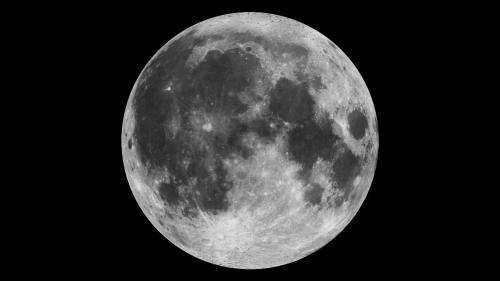
Improved age data for the Moon suggests that it is much younger than previously believed according to scientists presenting at a Royal Society discussion meeting entitled Origins of the Moon this week (23 September). Professor Richard Carlson of the Carnegie Institution of Washington will say that Earth's Moon is more likely between 4.4 and 4.45 billion years old rather than 4.56 billion years old, as previously thought.
The young age for the Moon implies an origin by a late giant impact into Earth with potentially big consequences for the Earth too.
Scientists have long studied lunar crustal rocks to try and estimate the age of the Moon. In the past obtaining accurate ages for lunar crustal rocks hasn't been easy for technical reasons. However as methods have improved, the ages of lunar crustal rock have begun to cluster not near 4.568 billion years, the precisely determined start time of Solar System formation, but between 4.36 and 4.45 billion years. Looking then at the Earth returns less clearly defined ages for Earth formation, but again, the ages tend to be less than 4.5 billion years.
Current models for planet formation assemble dust in the planetary nebula pretty quickly - where "pretty quickly" means a couple of million years. When assembled at this rate, the energy from violent collisions between planetesimals (small celestial bodies thought to fuse and form planets) and the heating caused by decay of radioactive elements causes even small planetesimals to undergo large-scale or complete melting. Through this melting process, iron metal segregates to the centre of the planetesimal and most of the volatile elements move to the atmosphere. When this chemical differentiation occurs on a small planetesimal, the planetesimal does not have enough gravity to hold on to its atmosphere, so it escapes into space. Earth is very depleted in volatile elements compared to the average composition of the Solar System, likely because it formed from differentiated planetesimals that had already lost their atmospheres.
Professor Carlson uses the example of the asteroid Vesta to explain the variety of approaches scientists have taken to estimating the Moon's age:
"If you asked the question 'How old is the asteroid Vesta?' the answer would be 4.565 ± 0.001 billion years. Scientists can state this so precisely because the global melting of the asteroid Vesta, as sampled by a group of meteorites known as eucrites, happened so quickly that the age was frozen in precisely in the rocks formed during this event. Furthermore, no later significant geologic events happened to disturb the age recorded by the rocks because Vesta is too small to retain enough interior heat to allow further melting/volcanism.
However, ask the same question of the Earth or Moon and you don't get a very precise answer. Earth likely took longer to grow to full size compared to a small asteroid like Vesta and every step in its growth tends to erase, or at least cloud, the memory of earlier events."
By comparing planetary ages in this way, scientists have concluded that Moon formation, which many believe to be the result of a very large impact into the proto-Earth, did not occur until about 4.4 to 4.45 billion years ago. The giant impact set the "age of the Moon" but also reset most (but not all) older ages that can be used to estimate the "age of the Earth'.
The most precisely determined age for the type of lunar crustal rock that is believed to form directly from the magma ocean that occurred during Moon formation is 4.360 ± 0.003 billion years. Over the last decade or so, two areas have been found on Earth that have crustal rocks/minerals with ages approaching this date. The first is an area where a few zircon grains were found in much younger sediment in Western Australia. The other is a group of rocks found along the shores of Hudson's Bay in Canada (the Nuvvuagittuq terrane). Other regions of very old Earth rocks (Isua Greenland, and the Acasta rocks in central Canada) are also beginning to show evidence of a major differentiation event on Earth around 4.45 billion years ago, so the possibility exists that we are now seeing the first crusts formed on both Earth and Moon after the giant impact.
Professor Carlson says:
"There are several important implications of this late Moon formation that have not yet been worked out, for example, if the Earth was already differentiated prior to the giant impact, would the impact have blown off the primordial atmosphere that formed from this earlier epoch of Earth history?"
Scientists will discuss a number of different moon forming theories at the Royal Society meeting with other topics including 'how does ongoing exploration of Mercury inform our understanding of the Moon?" and "Are the Earth and Moon isotopic twins?".
More information: royalsociety.org/events/2013/origin-moon/
Provided by The Royal Society