Mathematical model of a simple circuit in a chicken brain raises fundamental questions
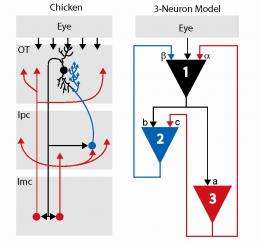
(PhysOrg.com) -- The Web site Neuroanthropology asks visitors to complete this quote, "One of the difficulties in understanding the brain is ...". In addition to the typical facetious remarks, such as "so few of us seem to have one" and "the damn thing is smart enough to realize what you are doing, and contrary enough to change the way it reacts just to spite you...," there are more serious ones, such as "... it's not a computer" and the methods we have available "are not enough accurate in saying how neural mechanisms correlates to behavior."
That's it in a nutshell. On the one hand, there are the individual nerve cells whose membrane depolarization is at the basis of everything and on the other hand, there's lyric poetry, serial murder and the calculus. In between there are hundreds of billions of nerve cells, with hundreds of trillions of connections. Scientists understand the bottom end and can say useful things about the top end, but getting from one end to the other is another story.
As they try, they argue continually and sometimes bitterly about levels of abstraction. If a model of the brain or part of the brain doesn't abstract at all, it is quickly snarled in unintelligible complexity. On the other hand, if it abstracts too much, it offers little insight into the organ it purports to represent.
In work published in the online edition of Physical Review E on November 25th, biophysicists and theoretical physicists at Washington University in St. Louis describe an elegant compromise. They devised a simple mathematical model that accurately represents a three-cell microcircuit in a chicken's brain. They then found, to their surprise, that they could collapse their model mathematically to one equation with two parameters, which are derived from but not the same as the strength of the connections between neurons (the synaptic strength) that neural models usually emphasize.
This mathematical exploration raises interesting questions about the living microcircuit in the chicken's tiny skull, such as how embryonic development wires it up in the first place. It may also explain why it is difficult to understand the brain by measuring the strength of its synaptic connections in the lab.
Lobster stomachs and chicken eyes
Neuroscientists have tried all sorts of different combinations of fidelity and abstraction in their efforts to understand the brain, but one fruitful approach has been to analyze a microcircuit consisting of a few neurons all of whose interconnections can be traced.
Microcircuits that have been analyzed in this way include the network that controls the chewing rhythm of a lobster's stomach (yes, it has teeth in its stomach), the reflex arc that allows a fruit fly to dodge a fly swatter and the timing network that controls the heartbeat of a medicinal leech.
Looking for a way to explain to the students in his Physics of the Brain class how delayed feedback produces complexity in a circuit, Ralf Wessel, Ph.D., associate professor of physics in Arts & Sciences, came up with a toy neural circuit simple enough to be stepped through time iterations at the blackboard. He used it to show his students that if there was feedback among the neurons, simple constant inputs could produce a long-period oscillation in their outputs.
Wessel then asked Matthew S. Caudill, Ph.D., graduate research assistant in physics, to create a computer model of the circuit so that it could be explored more thoroughly. As they worked with three-neuron microcircuit they realized it was very like one students in Wessel's neurophysiology lab were studying.
That circuit, which consists of three neurons and their feedback projections, has a simple task: to detect motion in the chicken's field of view. One neuron in the area called the optic tectum because it sits on the "roof" of the brain, sends axons to others in a knob of tissue called the nucleus isthmi. The neurons in the isthmi send projections back to the optic tectum, either directly to the neuron from which they got their input or back to the rest of the tectum (the crucial feedback loops).
There are similar microcircuits in the optical processing areas of reptilian and mammalian brains.
The microcircuit's behavior could be captured mathematically by three equations, each of which describes one neuron's output in terms of its inputs and parameters called synaptic weights, the standard way of expressing the strength of the connection between two neurons. Looking at the equations, Wessel and Caudill recognized that they could be reduced by algebraic substitution to one equation with two parameters (derived from the original five synaptic weights).
"It is as if," says Caudill, "the system of three neurons was reduced to one abstract neuron that does the same thing, follows the same rule, as the more complicated circuit. "
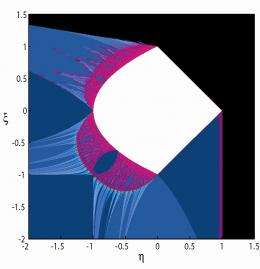
A Beautiful Parameter Space
This was an elegant outcome because all the possible solutions of an equation with two parameters can be mapped on a plane — which is what they did next, with the help of Zohar Nussinov, Ph.D., assistant professor of physics in Arts & Sciences.
When the output of the abstract neuron is mapped in the parameter space it looks like a colorful angelfish swimming in a black ocean.
"The blue regions are where the output is oscillating, the large white region is where it just goes to a steady value, and the black region is where the output diverges, or steadily increases," says Caudill. "The fans are areas where the period of oscillation changes quickly from one point to the next."
If you zoom in on the fans, Nussinov adds, the parameter space reveals more structure. Each time you zoom in, even more structure appears.
But what does this mean for the chicken? Why might an animal want a circuit to be stable most of the time but oscillate at others?
Weakly electric fish, says Wessel, have neural networks whose output oscillates in response to stimuli that affect the animal's whole body but is constant when the fish is touched in one spot (as it might be by the fin of a prey fish). The change in output allows the fish to distinguish instantly between global and local touch.
But Wessel is most interested in the way the work de-emphasized individual synaptic weights — even to the point of suggesting that focusing on these weights has gotten in the way of understanding brain function.
"Imagine you're an embryo and your brain is growing, he says, and the synaptic weights are being established. The brain doesn't need to establish the synaptic weights for each individual synapse in isolation. What it needs to establish is the combination of weights." But then he adds ruefully, "although how it would do that is not clear."
The finding also suggests why it might be difficult to achieve insight into neural circuits in the lab. Neurophysiologists probing neuronal circuits by inserting a glass electrode in one neuron, stimulating it, and recording the electrical activity of another neuron are likely to be baffled by physiological variations from animal to animal. The chicken microcircuit suggests that to understand a neural circuit they would have to measure combinations of synaptic weights. But the catch is they can't know which ones to measure unless they already know the rule the circuit follows.
What do the three scientists, who have simplified their microcircuit to the equivalent of one neuron, think of the Blue Brain project, an attempt funded by the Swiss government to create a synthetic brain by the brute force method of simulating roughly a million biologically realistic neurons with the help of a supercomputer?
Wessel says that perhaps the microcircuit is the orange clownfish Marlin and the Blue Brain is the blue regal tang Dory and together they are searching for Nemo: an understanding of how consciousness emerges from the soggy three-pound mass of nerve and glial cells we call the brain.
As you can tell, he has young children.
Provided by Washington University in St. Louis (news : web)