The cochlea's spiral shape boosts low frequencies
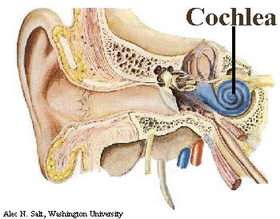
The next time someone whispers in your ear, think "cochlea." The cochlea is the marvelous structure in the inner ear that is shaped like a snail shell and transforms sounds into the nerve impulses that your brain can process and interpret. You may remember learning about it in elementary school anatomy.
This critical hearing organ consists of a fluid-filled tube about a cubic centimeter (three hundredths of an ounce) in volume. For decades, hearing experts thought that its spiral shape was simply an efficient packing job and its shape had no effect on how it functions. But a recent study headed by Vanderbilt mathematician Daphne Manoussaki calls this conventional wisdom into question. She and her colleagues, Richard Chadwick and Emilios Dimitriadis of the National Institutes of Health, have created a mathematical model of the cochlea that finds the spiral shape acts to enhance the low frequency sounds that we use to communicate with one another. They published the results recently in the journal Physical Review Letters.
If the new model is correct, then the cochlea is more sophisticated than researchers have thought. "This would indicate we need to take a step back from the cell biology and see how the cochlea works as an integrated system," says Karl Grosh, who studies the ear's structure at the University of Michigan in Ann Arbor. "The more we understand how the cochlea works, the more success we will have in building signal processing systems that mimic its auditory characteristics - an important aspect in designing cochlear implants and analog cochlear processors." According to the National Institute on Deafness and Other Communications Disorders, about 59,000 people have received cochlear implants worldwide and about 250,000 are potential candidates.
Manoussaki is an assistant professor of mathematics and her main interest is modeling cell movements. She got involved in studying the cochlea inadvertently. After she finished her doctoral degree from the University of Washington, she was looking for work. At NIH, Chadwick was looking for someone with computer skills. He saw her website, was impressed by the computer model of blood vessel formation that she had created and offered her a job as a visiting fellow. That is where she got involved in the workings of the inner ear.
"I knew nothing about cochlea mechanics and I think that was to my advantage," Manoussaki says. "I looked at this organ that was shaped like a snail but that everyone was modeling as if it were a straight duct and I asked the obvious question."
Chadwick informed her that it was well established that the spiral shape did not affect the way that the cochlea functions. In order to motivate her, however, he proposed that they review the papers that came to this conclusion. So that is what they did, one paper after another. It took them more than two years but they finally concluded that none of the existing proofs were persuasive.
That realization led them to develop a mathematical model of the cochlea that included its helical structure. Their first model, which portrayed the cochlea as a helix of constant radius, did not show that the shape had any effects. At the end of her fellowship, Manoussaki returned home to Greece. In 2004, as she was preparing to return to the United States, she reconnected with her collaborators and began working on the problem once again.
This time they developed a more sophisticated model. When sound waves enter the ear, they strike the ear drum and cause it to vibrate. Tiny bones in the ear transmit these vibrations to the fluid in the cochlea, where they travel along the narrowing tube that winds into a spiral. The tube is divided into two chambers by an elastic membrane that runs down its length. The mechanical properties of this "basilar" membrane vary from very stiff at the outer end and becomes increasingly flexible as the chambers narrow. These changing properties cause the waves to grow and then die away, much as ocean waves get taller and narrower in shoaling water. Different frequency waves peak at different positions along the tube. Hair cells sitting on the basilar membrane sense these motions by bending against the membrane and produce electrical signals that feed into the auditory nerve. Hair cells near the large end of the cochlea detect high-pitched sounds, such as the notes of a piccolo, while those at the narrow end of the tube detect lower frequency sounds, like a the oompah of a tuba.
This basic frequency sorting works in the same fashion whether the cochlear tube is laid out straight or coiled in a spiral. That observation, in fact, was the major reason that the researchers studying cochlear mechanics concluded its shape didn't matter. Manoussaki's model comes to the same conclusion, but her calculations also reveal that the spiral shape causes the energy in the waves to accumulate against the outside edge of the chamber. She likens this to the "whispering gallery mode" effect where whispers traveling along curved walls of a large chamber can remain strong enough so they can be heard clearly on the opposite side of the room.
This uneven energy distribution, in turn, causes the fluid to slosh higher on one side of the chamber, forcing the basilar membrane to tilt to one side, the direction to which the hair cells are most sensitive. The effect is strongest in the center of the spiral, where the lowest frequencies are detected. The researchers calculate the sensitivity increase can be as much as 20 decibels. That corresponds to the difference between the ambience of a quiet restaurant and the noise of a busy street.
It could easily make the difference between understanding that whisper in the ear or not.
Source: Vanderbilt University, by David F. Salisbury