Teleportation just got easier—but not for you, unfortunately
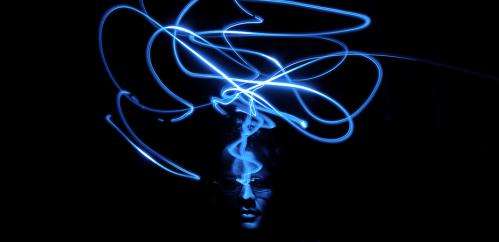
Thanks to two studies published in Nature last Thursday, the chance of successful teleportation has considerably increased. Which is a good thing, right?
Whether or not you've ever been on a long-haul flight, you've probably fantasised about being able to magically disappear from one place and reappear in another. And a natural question for a physicist is whether there is any way to achieve this in practice.
In fact, something known as "quantum teleportation" became a reality in 1997. This first demonstration was for particles of light (photons). Since then, physicists have also applied teleportation to other very small things, for example single atoms.
So when can we expect to just teleport ourselves to our chosen destination? You might want to sit down for this.
The first step to teleporting a person is measuring and recording the position, direction of motion and energy of every particle in the body, which would require more data storage than will ever be available – much, much more.
In fact, a conservative estimate would mean you'd need about 1022 gigabytes (1 followed by 22 zeros) of hard drive space. That's a stack of hard drives about 20 light-years tall.
Proxima Centauri, the nearest star to Earth other than the sun, is around four light-years away.
Worse, we have no method to even make these measurements, let alone reconstruct a person based on the data. So we can forget about teleporting people.
Knowing enough – but not too much
What about something really simple – such as a single particle? How about an atom, or a photon? How can these be teleported?
The problem here was thought to be the Heisenberg uncertainty principle, a cornerstone of quantum mechanics that limits what you can know.
It might sound counter-intuitive, but if you try to measure the position of a single atom you will change its velocity. If you find out exactly how fast it is moving, then you won't know where it is.
The problem is, if you want to teleport a particle, this is precisely the information you want to measure and transmit.
A physicist would call this information the "state" of the particle. If you're not allowed to measure the complete state of the particle, teleportation looks impossible.
So the key to teleportation is not knowing too much. As long as the measurements that you make do not reveal the position or velocity, then you have a loophole that allows you to circumvent the uncertainty principle.
What if you could disturb the particle before you measure it, so you never know its state, and then subtract off that disturbance at the other end to recreate the original state of the particle?
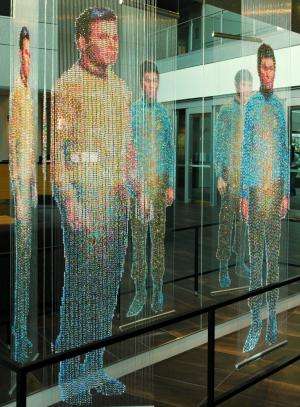
This was the breakthrough realisation that American physicist Charles Bennett had in 1993. The key was to disturb the particle you want to teleport in a particular way. You can do this by using a pair of quantum-entangled particles.
These particles are linked to each other so that if you measure the state of one of the entangled pair, you learn about the state of the other half of the pair.
Alice and Bob
In the standard description of teleportation, Alice is teleporting something to Bob. Alice uses one of the entangled particles to measure the state of the input particle. She records what she measures and sends the information to Bob.
Bob can't tell what the state of the particle was, because the entanglement used in the measurement hides the true nature of the state.
What Bob can do, however, is use the information from Alice to modify the state of the other entangled particle. In this way he can recreate the exact state of the particle Alice originally measured.
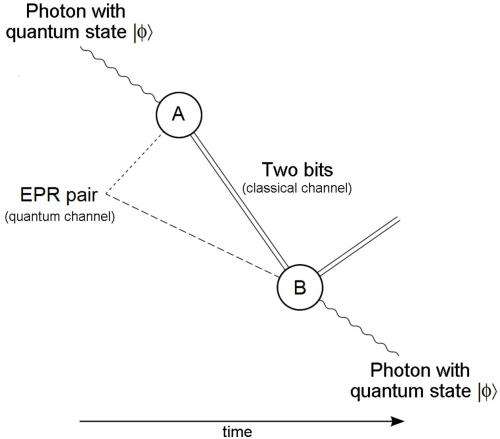
This is how quantum teleportation works. Most photon experiments teleport over a metre or so inside a lab, although there has recently been a demonstration over 143km in the Canary Islands.
A sense of security
It turns out that quantum teleportation is not just a good party trick. The nature of the communication between Alice and Bob in this system is pretty interesting.
The information that Alice measures and sends to Bob cannot be used to recreate the input state without the other entangled particle. That means Eve the eavesdropper can't spy on Alice's measurement and get the information for herself.
The entangled pair is unique, so only Bob can recreate the original state. Immediately you have a technique for secure communication.
If you encode information in your particles, measure them with one part of an entangled state and then send the information to Bob, you have cryptography that is made strong by quantum physics. You really can't crack it by any means, unless you have the other part of the entangled pair.
Reasons to be cheerful
Teleportation has many other uses in quantum information systems.
These are proposed methods for building computers and communication networks that use quantum mechanics as a core part of their functionality and have enormous potential to provide secure communications and high-speed computing.
The catch is that any time you want to move quantum information from one place to another in one of these systems, you can't just measure the information and send it to the next part of the process, since the measurement will destroy the information. Instead, you can teleport it.
Back to Nature
The two papers published together in this week's Nature show something very important.
Until now, teleporting photons of light using the method described above has been probabilistic, because you couldn't synchronise the arrival of the entangled photons with the arrival of the photon to be measured.
On the odd occasion when the photons aligned, the measurement would only work half the time. That means every time you try and teleport your information it will only work very occasionally – much less than 1% of the time.
If you have a lot of back-to-back-teleporting circuits in your quantum computer or quantum network, the chances of them all working together will become vanishingly small.
These two most recent experiments show deterministic quantum teleportation in two different systems so that the process is no longer probabilistic. Instead it can, in principle, work every time a photon is ready to be teleported.
One of the new studies – by researchers from Japan and Germany – shows how it is possible to teleport photons of light that are in the infrared spectrum, just below the wavelength visible to the human eye.
The other experiment – by researchers in Switzerland and Australia – demonstrates teleportation of microwave photons with a frequencies between 4 and 7 GHz.
Neither system is production-ready, in the sense that they are both just proof of principle experiments. Although the teleportation is no longer probabilistic, it is still not 100% efficient – a 40% chance of success in the case of the infrared system and 25% in the case of the microwave system.
Still, this is a vast improvement on less than 1% that was previously possible with photons. Long-haul flights will continue for some time yet, but the new experiments represent a milestone on the long road to building a functional quantum information system.
Journal information: Nature
Provided by The Conversation
This story is published courtesy of The Conversation (under Creative Commons-Attribution/No derivatives).