June 5, 2008 feature
'Squeezed' Light May Improve Gravitational Wave Detectors
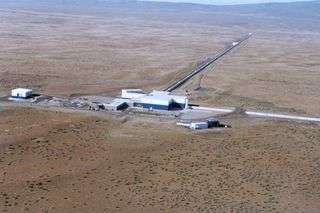
A research collaboration has taken steps toward improving the sensitivity of gravitational wave detectors, devices designed to measure distance changes as minute as one-thousandth the diameter of a proton. Scientists hope these detectors can one day further verify Einstein's theory of general relativity and even open a new window into the strange workings of the universe.
Gravitational waves, such as those emitted by extremely massive astronomical sources like neutron stars and black holes, can change the distance between two objects—even if that distance change is almost incomprehensibly small—by altering the shape of spacetime. Devices that can sense gravitational waves are among the most sensitive position meters ever built, but, right now, their abilities are inherently limited by quantum mechanics.
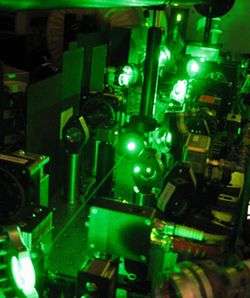
Laser interferometer gravitational wave detectors use laser light to measure the distances between mirrors. And light, being an electromagnetic (EM) field, is quantum in nature. EM fields contain quantum noise, fluctuations that make the beams less “sharp,” so to speak. Fluctuations mean that the physical parameters describing the beam are not fixed, but instead have built-in uncertainty or fuzziness, which limits the sensitivity of a gravitational wave detector.
The researchers in this study, from several institutions in the U.S. and Australia,* seem to have found a solution to this problem: using “squeezed” light. When light is squeezed, the fluctuations of one parameter, such as the light wave's amplitude, can be reduced at the expense of increased fluctuations of another parameter, such as its phase. The amplitude is a measure of the number of photons in the light beam, while the phase describes its location in time. Quantities corresponding to amplitude and phase are called quadratures, and quantum mechanics allows the uncertainty in one quadrature to be reduced as long as the uncertainty in the complementary quadrature increases.
In this case, the scientists squeezed one quadrature of the light, decreasing its quantum noise. While the noise of the other quadrature is necessarily increased, it is a quadrature they don't need to measure. By injecting the squeezed EM field in their prototype detector, the scientists were able to make it 44 percent more sensitive.
“The significance of this work is that it forced us to confront and solve some of the practical challenges of squeezed state injection—and there are many,” said Massachusetts Institute of Technology physicist Nergis Mavalvala, the team's leader.
“We are now much better positioned to implement squeezing in the kilometer-scale detectors, and catch that elusive gravitational wave.” she added.
Gravitational wave detectors work, in general, by interfering two laser beams that have traveled very long distances. The beams travel down two arms that form a 90 degree angle. While each arm is up to 4 kilometers in length, mirrors reflect the beams back and forth multiple times, effectively increasing the arm length to a few hundred kilometers. If a gravitational wave from deep space alters the length of each arm, the change to the laser interference pattern will be measurable.
Existing gravitational wave detectors are so sensitive that they have already approached the quantum limit. They include the two LIGO (Laser Interferometry Gravitational Wave Observatory) facilities in Washington and Louisiana, both operated jointly by the Massachusetts Institute of Technology and the California Institute of Technology; and Europe's largest gravitational wave detector, Virgo, located in Italy.
Discover the latest in science, tech, and space with over 100,000 subscribers who rely on Phys.org for daily insights. Sign up for our free newsletter and get updates on breakthroughs, innovations, and research that matter—daily or weekly.
The researchers say that the next generation of gravitational wave detectors, such as Advanced LIGO, the planned upgrade to both LIGO facilities, will boast some impressive improvements, but further advancements will have to rely on quantum techniques such as squeezing.
Citation: K. Goda, O. Miyakawa, E. E. Mikhailov, S. Saraf, R. Adhikari, K. McKenzie, R. Ward, S. Vass, A. J. Weinstein, and N. Mavalvala Nature Physics advance online publication, 30 March 2008 DOI:10.1038/nphys920
*Massachusetts Institute of Technology, Cambridge, Massachusetts; California Institute of Technology, Pasadena, California; The College of William and Mary, Williamsburg, Virginia; Rochester Institute of Technology, Rochester, New York; and The Australian National University, Canberra, Australia
Copyright 2008 PhysOrg.com.
All rights reserved. This material may not be published, broadcast, rewritten or redistributed in whole or part without the express written permission of PhysOrg.com.