On the Energy Trail: Researchers Find New Details Following the Path of Solar Energy During Photosynthesis
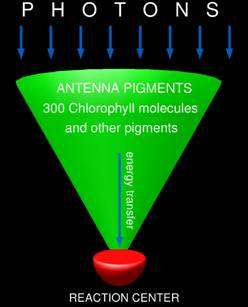
Imagine a technology that would not only provide a green and renewable source of electrical energy, but could also help scrub the atmosphere of excessive carbon dioxide resulting from the burning of fossil fuels. That’s the promise of artificial versions of photosynthesis, the process by which green plants have been converting solar energy into electrochemical energy for millions of years. To get there, however, scientists need a far better understanding of how Nature does it, starting with the harvesting of sunlight and the transporting of this energy to electrochemical reaction centers.
Graham Fleming, a physical chemist who holds joint appointments with the U.S. Department of Energy’s Lawrence Berkeley National Laboratory and the University of California at Berkeley, is the leader of an ongoing effort to discover how plants are able to transfer energy through a network of pigment-protein complexes with nearly 100-percent efficiency. In previous studies, he and his research group used a laser-based technique they developed called two-dimensional electronic spectroscopy to track the flow of excitation energy through both time and space. Now, for the first time, they’ve been able to connect that flow to energy-transferring functions by providing direct experimental links between atomic and electronic structures in pigment-protein complexes.
“To fully understand how the energy-transfer system in photosynthesis works, you can’t just study the spatial landscape of these pigment-protein complexes, you also need to study the electronic energy landscape. This has been a challenge because the electronic energy landscape is not confined to a single molecule but is spread out over an entire system of molecules,” Fleming said. “Our new 2D electronic spectroscopy technique has enabled us to move beyond the imaging of structures and to start imaging functions. This makes it possible for us to examine the crucial aspects of the energy-transfer system that enable it to work the way it does.
In a paper published by the Biophysical Journal, Fleming and his group report on a study of the energy-transferring functions within the Fenna-Matthews-Olson (FMO) photosynthetic light-harvesting protein, a pigment-protein complex in green sulfur bacteria that serves as a model system because it consists of only seven well-characterized pigment molecules. The paper, entitled “Visualization of Excitonic Structure in the Fenna-Matthews-Olson Photosynthetic Complex by Polarization-Dependent Two-Dimensional Electronic Spectroscopy,” was co-authored by Elizabeth Read, along with Gabriela Schlau-Cohen, Gregory Engel, Jianzhong Wen and Robert Blankenship.
“The optical properties of bacteriochlorophyll pigments are well understood, and the spatial arrangement of the pigments in FMO is known, but this has not been enough to understand how the protein as a whole responds to light excitation,” said Read. “By polarizing the laser pulses in our 2D electronic spectroscopy set-up in specific ways, we were able to visualize the direction of electronic excitation states in the FMO complex and probe the way individual states contribute to the collective behavior of the pigment-protein complex after broadband excitation.”
Fleming has compared 2D electronic spectroscopy to the early super-heterodyne radios, where an incoming high frequency radio signal was converted by an oscillator to a lower frequency for more controllable amplification and better reception. In 2D electronic spectroscopy, a sample is sequentially flashed with light from three laser beams, delivered in femtosecond timescale bursts, while a fourth beam serves as a local oscillator to amplify and phase-match the resulting spectroscopic signals.
“By providing femtosecond temporal resolution and nanometer spatial resolution, 2D electronic spectroscopy allows us to simultaneously follow the dynamics of multiple electronic states, which makes it an especially useful tool for studying photosynthetic complexes,” Fleming said. “Because the pigment molecules within protein complexes have a fixed orientation relative to each other and each absorbs light polarized along a particular molecular axis, the use of 2D electronic spectroscopy with polarized laser pulses allows us to follow the electronic couplings and interactions (between pigments and the surrounding protein) that dictate the mechanism of energy flow. This suggests the possibility of designing future experiments that use combinations of tailored polarization sequences to separate and monitor individual energy relaxation pathways.”
In all photosynthetic systems, the conversion of light into chemical energy is driven by electronic couplings that give rise to collective excitations - called molecular or Frenkel excitons (after Russian physicist Yakov Frenkel) - which are distinct from individual pigment excitations. Energy in the form of these molecular excitons is transferred from one molecule to the next down specific energy pathways as determined by the electronic energy landscape of the complex. Polarization-selective 2D electronic spectroscopy is sensitive to molecular excitons - their energies, transition strengths, and orientations - and therefore is an ideal probe of complex functions.
“Using specialized polarization sequences that select for a particular cross-peak in a spectrum allows us to probe any one particular electronic coupling even in a system containing many interacting chromophores,” said Read. “The ability to probe specific interactions between electronic states more incisively should help us better understand the design principles of natural light-harvesting systems, which in turn should help in the design of artificial light-conversion devices.”
Source: Lawrence Berkeley National Laboratory