December 4, 2014 report
Origin of the Eukaryotic cell: Part I - How to train your endosymbiont
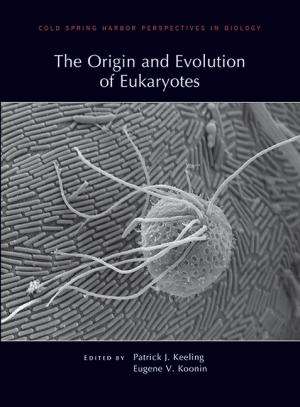
(Phys.org)—"The origin and evolution of eukaryotes" is a tale that has yet to be told. At this point in time, it exists only as the title of a fascinating new compendium that has just been produced by the Cold Spring Harbor Press. While this volume is some 400 pages long, there is a palpable feeling among its authors that if they could somehow just compact it into a few pages, a figure, and perhaps even a token energy equation, then what is now just a diffuse description would become the explanation we seek—maybe even without any new discoveries or information.
The nearest the book comes to providing that holy explanation is undoubtedly the chapter by Thomas Cavalier-Smith dealing with what he likes to call the "Neomuran Revolution," which we will describe below. At its heart, what Cavalier-Smith offers is a sweeping tree of life that has continued to grow with the abundant fertilizer he has added over the last several decades. Incidentaly, we should add that one of the best ways to expedite your ascendance to prominance in the origin of life business is to simply expand your last name with - Smith. Both Graham Cairns-Smith and John Maynard Smith were masters at this and their work continues to shape the field.
Instead of the typical three domains of life—bacteria, archaea (archaebacteria), and eukaryotes—that many now presume, Cavalier-Smith maintains that the latter two form a sister clade (neomura), whose origins refute the now popular theory that eukaryotes originated by merging an archaebacterium with an alphaproteobacterium. The defining feature of clade neomura, he suggests, is the loss of the murein wall in certain posibacteria—his world for a refined class of gram-positive bacteria. This evolution, taking place ~1.2 Gy ago, would have enabled a more flexible cell complete with novel modes of cell division, DNA segregation, phagocytosis, and ambulation.
The departure of some of Cavalier-Smith ideas from the current mainstream view takes root with the so-called "hydrogen hypothesis" which we discussed here fairly recently. When extended to its logical conclusion, this theory presumes that the above-mentioned merger that enabled the aquisition of a mitochondrial precursor (the alphaproteobacterium) by a methanogen (the archaeobacterium) precipitated the development of a complex nucleus through subsequent gene transfer from the mitochondrion, along with the prompt establishment of the other modern accoutrements of the eukaryotic cell.
Among the questions most poignately raised by the hydrogen hypothesis are what was the actual genetic contribution to the eukaryote, and when exactly did this purported mass mitochondrial gene exodus take flight across the Red Sea cytoplasm to nucleus. Some clues come from the chapter by David Moreira and Philippe Deschamps, which like others, looks more broadly at the question of endosymbiont acquisition. In particular, they do a phylogenetic analysis of plastids, the photosynthetic apparatus possed by some eukaryotes. It is now widely believed that photosynthesis began in eukaryotes by endosymbiosis with cyanobacteria. This event would have resembled the previous mitochondrial mergers only it is now estimated to have ocurred roughly 500 million years later.
The book is not without occaisional claims of "settled science," as a subsequent chapter by Michael Gray indicates. This is probably forgiveable because he gets around to making some good points. Within his unseen circle of confidence he offers that while the DNA of mitochondria (mtDNA) is "unquestionably the remnant of a-proteobacterial genome," he reveals that only 10%-20% of the mitochondrial proteome is demonstrably alphaprotobacterial in origin. Gray's "pre-endosymbiont hypothesis" suggests that the host cell that accomodated the new symbiont may have already had genes for several of the many non-energy generating functions found in a contemporary mitochondrion already esconced away in a membrane-bound metabolic organelle which he calls a premitochondrion. Just to clarify, this couterflow of gene products from an extant endogenous compartment to the new symbiont would exist in tandem with a background flux of new symbiont genes encoding proteins for the symbiont (which must then somehow acquire organelle localization signals) to the nucleus.
We might call this hypothetical endogenous premitochondrial organelle a "dark endosymbiont" in the spirit of the dark matter that is inferred to exist, or must have existed at some point in the past in our universe. In their chapter on plastids, Moreira and Deschamps note that genetic analysis has previously led others to propose similar kinds of cryptic organelles that are no longer visible in the cell. While these kinds of things are certainly possible, Cavalier-Smith is quick to point out the limitations of what he refers to as molecular myopia and mythical molecular clocks. Without some other kind of analysis to back it up, even if it is just protein conformation comparisons for example, molecular sequence alone invariably leads to ambiguous or even contradictory conclusions. As the gaps in knowledge of the origins of the eukaryotic cell are now being rapidly filled in, it seems that it is becoming more difficult to accept any one part of any broad proposal for a definitive tree of life without accepting the whole thing.
One good example of how to quickly get confused when favoring a gene-centric approach at the expense of paleontologic or structural organelle-level analysis is with ribosomes. While horizontal, lateral and verticle gene flow among bacteria, archaea, and eukaryotes are constant confusers across species, the ribosomal RNA and riboproteins that exist in several unique cell-trafficking or inheritance scenarious in the organelles of single cell can be even more confounding. Cavalier-Smith has offered several ideas here to explain the size changes that have occured in the evolution of particular ribosomal subunits found in mitochondrial and nuclear DNA, as well as the localization motifs they have.
In a follow up post we will address some of the main structural features of cells that have been critical in defining the evolutionary path taken by eukaryotes. Chief among these are the different ways that various kingdoms of life make their membranes, cytoskeletons, and cilia. We will also explore the precursors to spliced introns, centrioles, meisosis, recombination, sex, and perhaps multicelluarity. The finer scale structure of golgi, and the bacterial precident for mitochondrial cristae should also be included. Finally we will look at some attempts to root major eukaryotic developments in terms of the ecological background of Earth, its oxygenation, and maybe even a glaciation event or two.
More information: The Origin and Evolution of Eukaryotes: cshperspectives.cshlp.org/cgi/ … lution_of_eukaryotes
Part 2 of the story is here: phys.org/news338016128.html
© 2014 Phys.org