Biomarkers of the deep
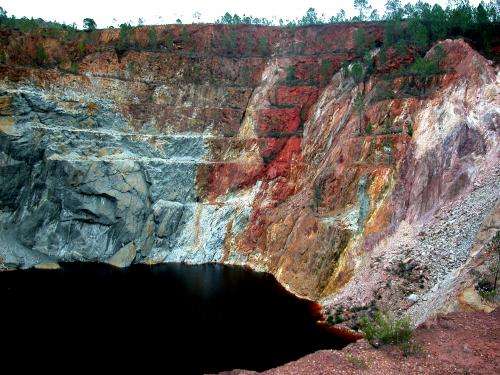
Tucked away in the southwest corner of Spain is a unique geological site that has fascinated astrobiologists for decades. The Iberian Pyrite Belt (IPB) in Spain's Río Tinto area is the largest known deposit of sulfide on Earth, and for decades it has been a field-site for scientists studying chemolithotrophic microbes.
Many of these unique organisms are thought to survive independently of the Sun and instead gather the energy they need to live from the chemical imbalance of minerals. Organisms with this ability could stand the best chance of surviving in environments on other worlds, like the deep subsurface of Mars.
"The Río Tinto mineralogy is dominated mainly by iron and sulfur minerals such as hematite and jarosite, both already discovered on Mars," said Victor Parro Garcia, head of the Molecular Evolution Department at the Centro de Astrobiología (CAB) (INTA-CSIC) in Spain. "These minerals are the consequence of the pyrite (FeS2) oxidation, a process highly accelerated by iron oxidizing microbes to obtain energy for their metabolisms."
In the Rio Tinto, these microorganisms don't need the Sun to survive. To grow, all they need is iron, carbon and nitrogen CO2 and N2 in the atmosphere, and some salts from the water for growth.
"They have very simple nutrient requirements," said Parro Garcia, a co-author on the recent study. "In summary, the Iberian Pyrite Belt subsurface is an excellent analogue for a potential Martian subsurface habitat because of its mineralogy and the variety of anaerobic metabolisms that can occur there."
The Red River
Legend has it that the region surrounding Spain's Río Tinto River was once the location of King Solomon's mines. Today, the region is still famous for its mining history, but astrobiolgists have also found a treasure-trove of data that explain the limits to habitability on Earth. Due to its incredibly high iron content, the acidic river flows like red wine through a multicolored and rocky landscape that at sometimes resembles an alien world.
The Río Tinto has an average pH of 2.3, which is acidic enough to eat metal. For years, scientists believed that industrial pollution from 5,000 years of mining in the region was responsible for this harsh environment. However, when astrobiologists began searching for life in the river, they found that microorganisms also played a role in maintaining the acidity through a process known as acid rock drainage. This naturally produces acid runoff when microbes interact with sulfide from the IPB.
"The water table and the high temperatures all year around favor the growth of chemolithotrophic bacteria, which exist in high amounts in the river and probably in underground waters," explained Ricardo Amils in a 2002 interview with Astrobiology Magazine.
Amils has been studying the Tinto river for decades as director of the laboratory of applied microbiology at the Center for Molecular Biology (CBM) at the Autonomous University in Madrid.
"The most important characteristics of the system—sulfuric acid and high concentrations of ferric iron—are products of chemolithotrophic activity using pyrite and other sulfidic minerals."
In the early 2000's, NASA joined with CAB to explore habitats for life beneath the Earth in the region of the Rio Tinto. The project was known as the Mars Analog Research and Technology Experiment (MARTE), and for more than a decade the project has been using the Rio Tinto as an analog site for testing equipment that could be used on future Mars missions. This includes remote drilling techniques that provide scientists with access to the deep subsurface. Previous studies were mostly focused on testing the equipment, but now a team of scientists has performed a thorough investigation of the microorganisms collected in the cores.
The team drilled into the ground in different locations and to depths ranging from just 5 meters down to over 166 meters. Every time the drill bit moved one meter, a sample was collected and multiple tests were performed, including: DNA extraction, cultivation, ATP activity, Microscopic analysis, or Geochemistry analysis.
"In total, more than 200 samples were collected for biology," said Parro Garcia.
Previous work identified specific species of organisms that survive in the subsurface at Rio Tinto. The new study focuses on deeper drill cores collected by the MARTE project, and paints a more complete picture of the diversity and interaction of microorganisms that thrive in this acid-stained earth.
Spitting out Acid Underground
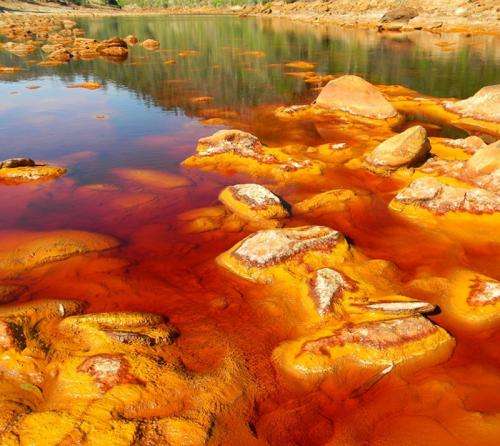
The deep subsurface may be one of the best environments in which to study life's potential on other rocky worlds in the Solar System. Deep below ground, specific types of microorganisms have access to resources that allow them to live independently of light from the Sun or the rest of the biosphere at the surface. The thick layers of rock and soil also provide protection from environmental stresses like temperature, pressure and radiation that could otherwise kill them. The deep subsurface of the Rio Tinto is particularly interesting, because can create the acidic niches that pushes life even closer to the limit of habitability, and because is the responsible for the acidic waters of the river.
The team looked at sections of a drill core that started at 90 meters below the surface and continued down to 164 meters. They were particularly interested in the biological processes happening in sections of the core where conditions were anaerobic (meaning oxygen is not present).
Samples of rock were collected from inside the drill cores and either analyzed immediately on site or frozen and brought into the lab. In their analysis, the team used a wide range of methods and equipment to study the geochemistry of samples, detect complex organic matter, and identify microbial communities.
In terms of the geochemistry, they focused on anions that can be used in microbial metabolism (think vitamins in your bathroom cupboard). This included things like nitrate, sulfate, iron and organic acids like acetate and formate. The concentrations of all these biologically-important anions throughout the length of the core helped them determine which microbes were living at different levels in the subsurface, and how the communities were interacting with each other.
To identify the microbes, the team collected and analyzed DNA from samples throughout the length of the core. They also used life detection chips that contain individual antibodies that react with specific biomolecules.
"At least 40 of the samples were also analyzed by a Life Detector Chip (LDChip) containing 200 antibodies," Parro told Astrobiology Magazine.
By exposing a sample to each of the antibodies, you can test the types of molecules that are present simply by looking at the antibodies that react. Then, you can check and see which organisms match up with which biomolecules.
The team also stained samples with different types of DNA and RNA-binding dyes that help determine whether or not the cells are actually alive and working, or if they are just left-over material from cells that died long ago.
In the samples, there was evidence for numerous types of microorganisms, including methanogens, iron-respiring bacteria, ferric iron reducers, methane oxidizers and denitrifying bacteria. In some parts of the core, aerobic microorganisms were also found (e.g. microbes that live in the presence of oxygen). This showed that not all of the deep subsurface environments were anaerobic.
All of the data from the microbe tests was then correlated with the geochemical data to create a model of the IPB subsurface ecosystem that was broken into three parts.
The first section is a zone where some oxygen is present in small pockets. Here, organisms get the energy to survive by oxidizing methane, iron and sulfur. Outside of the oxygen-rich areas, anaerobic organisms perform denitrification and methanogenesis..
The second zone is completely anaerobic, and the ecosystem thrives in a similar fashion to the aerobic areas higher up.
The third and deepest zone contains fractured rock that allows more water to flow into the system, increasing the availability of oxygen once again. This influx of resources means that communities are more metabolically active. Like zone one, pockets of both aerobic and anaerobic environments exist, allowing for the various types of metabolism to occur.
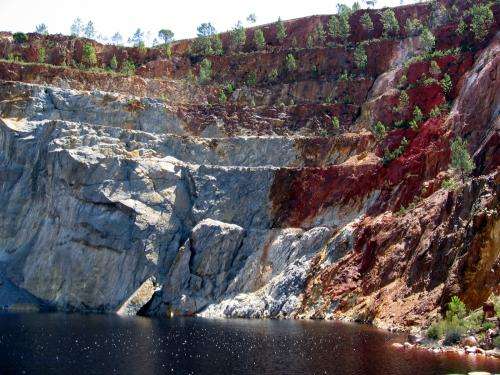
"These results, together with measures of the geochemical parameters in the borehole, allowed us to create a preliminary scheme of the biogeochemical processes that could be operating in the deep subsurface of the Iberian Pyrite Belt, including microbial metabolisms such as sulfate reduction, methanogenesis and anaerobic methane oxidation," the researchers concluded in their paper.
All together, the team showed that the deep subsurface at the Rio Tinto contains a very diverse community of microorganisms, heterogeneously distributed in multiple microniches, some of them acidic and other at circumneutral pH.
"I did not expect such diversity in such a hard rocky cores," said Parro. "One realizes that life is really tough and colonizes any accessible niche."
Next, the team will drill even deeper below ground and add tests for even more types of microbial metabolism that could be occurring. In a new effort, they have already drilled down to 612 meters as part of the Iberian Pyrite Subsurface Life experiment (IPBSL), led by professor Ricardo Amils at Centro de Astrobiología (INTA-CSIC), and funded by a European Research Council Advanced Grant.
As the study goes deeper, environments with oxygen will likely become less and less available, favoring anaerobic metabolism. Studying these samples will not only provide an opportunity to better-explain the Rio Tinto environment, but could also bring astrobiologists closer to understanding possible survival strategies for life on Mars.
"Other anaerobic metabolisms do not need inputs from the exterior (that is organic matter from photosynthesis), such as acetogenesis (the production of acetic acid instead of methane), nitrate reduction or methane consumption by certain anaerobic microbes," said Parro. "These are highly relevant for a potential Martian biota."
Hunting for Exo-biomarkers
Another outcome of the study is in showing that some of the techniques used to detect the signatures of microbial life in the Rio Tinto subsurface could be useful in future exploration beyond Earth. The multiple techniques provide redundancy, and none of them require microbes to be cultured in a laboratory. Samples can be collected in the field and immediately analyzed, and the study could help scientist integrate these techniques into automated robotic missions.
One such instrument is the Sings Of Life Detector (SOLID) in development at CAB. This automated system could collect samples of dust, regolith or rock on Mars, and then extract organic matter, suspend it in solution, filter it, and expose it to a life detection chip full of antibodies to identify biomolecules.
The more we learn about microbial metabolism in the subsurface, and the multitude of ways that organisms are able to eek out a living on the limited resources available there, the closer we get to finding molecules that we could search for in order to identify life in the Solar System. Parro already has an idea of what he would hunt for in the subsurface of Mars.
"I would search for biomolecules (peptides, nucleic acids, polysaccharides) and cell or biofilm debris in subsurface samples with our Life Detector Chip immunosensor and the SOLID (Signs Of Life Detector) instrument."
Provided by Astrobio.net
This story is republished courtesy of NASA's Astrobiology Magazine. Explore the Earth and beyond at www.astrobio.net .