Researchers Test the Physics of Star Formation in the Lab
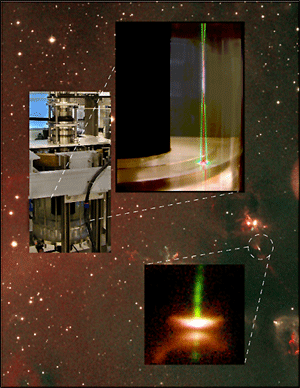
The formation of stars and planets remains one of the big questions in astrophysical science. Currently, scientists do not understand the required conditions and the accretion, or matter collection process, involved in star and planet formation. There are contentious debates about whether hydrodynamic turbulence is responsible.
The Magnetorotational Instability (MRI) experiment at the Princeton Plasma Physics Laboratory (PPPL) in collaboration with the Astrophysical Sciences Department of Princeton University is shedding light on this mystery. Results published in the November 16th issue of Nature show that it is virtually impossible for hydrodynamic turbulence to generate sufficiently effective accretion to form stars and planets. The U.S. Department of Energy, NASA, and NSF are funding the work jointly.
"The Earth must have sufficient angular momentum so that it does not fall into the Sun under the influence of gravity. We also know that galaxies and solar systems have a preferred direction of rotation. Consequently, matter forming these systems must also have had net angular momentum, which must have been overcome by gravity for the matter to coalesce," said Hantao Ji, the lead author of the Nature paper. "The angular momentum prevents matter from falling into the star directly, so an accretion disk is formed, which consists of matter losing its angular momentum and swirling into the core of the star. For example, when our Sun was formed, the accretion process must have been very efficient in casting off angular momentum because most of the material comprising our solar system ended up in the Sun." Since angular momentum cannot be created or destroyed, it must flow outward through the disk as the accreting mass flows inward. But how does this happen?
Star formation occurs in deep space and therefore, while accretion disks are seen, the details of the accretion process cannot be discerned except in theoretical models and computer simulations. The Princeton project's primary mission is to test the plausibility of a 1991 theory that indicates the magnetorotational instability, a disruptive plasma process, plays a major role in accretion. The elimination of hydrodynamic turbulence as a mechanism for accretion, makes it much more likely that magnetorotational instability is responsible.
Matter in an accretion disk is composed of plasma, dust, and other materials. However, the MRI experiment does not use these materials. Ji and Jeremy Goodman, the primary collaborator from the Princeton University Astrophysics Department and also a co-author of the Nature paper, came up with a way to physically simulate an accretion disk with material "standing in" for the plasma, dust, and other materials.
The system consists of two concentric cylinders, each 28 centimeters in length, free to rotate independently about a common axis. The inner cylinder has a radius of 7.1 centimeters and is made of steel, and the outer cylinder has a radius of 20.3 centimeters and is made of plastic to allow visual inspection. The inner and outer cylinders rotate independently in the same direction, but at significantly different speeds, 1200 rpm and 160 rpm, respectively, as reported in the paper. What made this project a significant engineering challenge is the requirement to have two rotating disks at each end of the cylinders. The disks must be driven at different speeds by separate motors through six concentric pipes in order to achieve the required rotation patterns of the fluid.
For the experiments currently reported in Nature, the space between the cylinders was filled with water. Water cannot carry a significant electrical current or interact with a magnetic field and therefore cannot display magnetorotational instability, but according to nonmagnetic theories of accretion disks, should have become turbulent anyway with fast enough spinning.
Future experiments are planned in which the space between the cylinders will be filled with a liquid metal chosen because it is easy to maintain and interacts with the magnetic field in ways similar to plasma. The researchers have chosen a mixture of 67 percent gallium, 20.5 percent indium and 12.5 percent tin.
Future experiments will be conducted with and without a magnetic field parallel to the axis of the cylinders. Computer simulations of the experiment predict that when a strong magnetic field is applied to the rotating liquid metal, magnetorotational instability will cause angular momentum to be transferred from the inner cylinder toward the outer cylinder, resulting in an increase in measured torque between the cylinders. This result would further support the hypothesis that magnetorotational instability is responsible for the transport of angular momentum in accretion disks, and hence for star formation.
Accretion disks also form around massive black holes in the center of many galaxies and in binary star systems. Results from the PPPL experiments will help astrophysicists better understand these phenomena. Understanding transport phenomena in plasmas is important for basic plasma physics in general, and for fusion plasmas in particular.
Source: Princeton University